4 What Does It Mean to be Human?
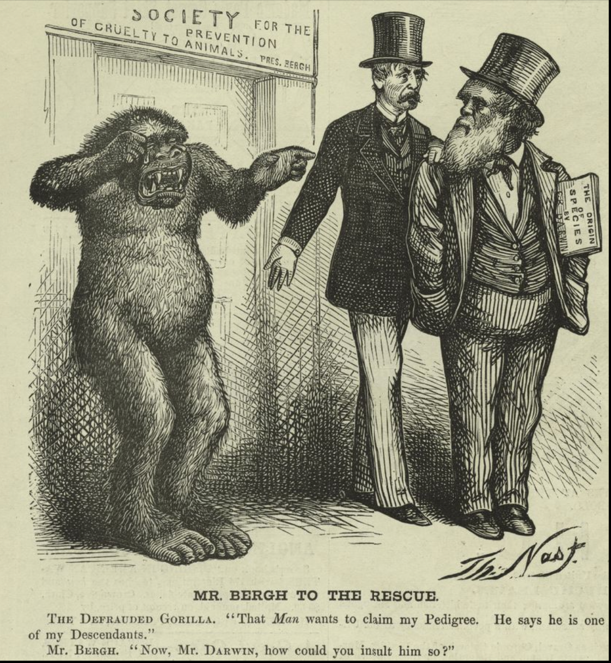
Since Darwin published his opus, On the Origin of Species by Natural Selection, in 1859, many have labored under a fundamental misconception—that humans are descended from contempoary gorillas, chimpanzees, or monkeys that populate the earth along with us. Darwin never claimed this. Neither have biologists since then. Are we a primate? Yes. Are we descended from gorillas, chimpanzees, or monkeys? No. We share a common ancestor with our fellow primates but that does not mean we “descend” from them. Other living primates have a different evolutionary path than humans.
In this chapter, you will explore how humans are related to other primates and what distinguishes us from our primate cousins. But first, it is important to understand the relationships between organisms, which is highlighted by the Linnean taxonomic system.
Constructing Relationships
Taxonomy is the field of biological classification that uses nested sets of categories of increasing specificity to illustrate relationships between organisms (Park 2014, 99). Prior to the availability of DNA data, comparative anatomy was used to establish relationships between organisms. Today, when suitable samples are available, DNA sequencing is used to establish relationships. Paleontologists and paleoanthropologists continue to use comparative anatomy to construct relationships among fossil species. A notable exception is with the fossil species Homo neanderthalensis and archaic Homo sapiens. In some instances, researchers were able to collect DNA samples to sequence.
But what does DNA sequencing tell us? Because DNA mutates at a known rate, scientists can determine how alike the sequences are. The more alike, the more recent the divergence from a common ancestor. This data can then be used to create evolutionary trees (Lewis 2016). Establishing these relationships demonstrates a unity to the natural world—an interconnectedness that is key to understanding how earth’s ecosystems work.
From philosophers such as Aristotle (384-322 BCE) to naturalists such as John Ray (1627-1705), people attempted to classify life, providing some order to the vast amount of knowledge that was being accumulated. The taxonomic system created by Carolus Linnaeus (1707-1778) in 1758 is the basis of the system still used by scientists today.
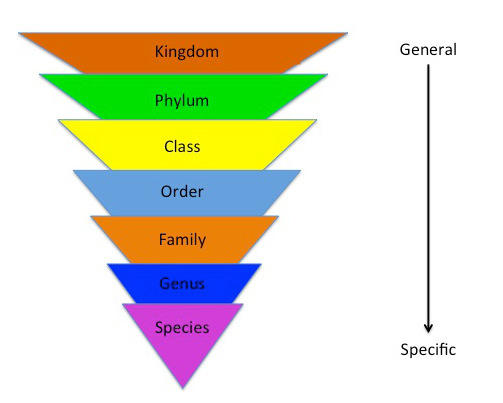
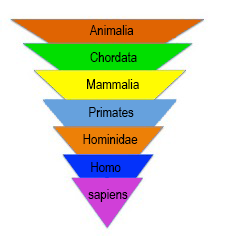
Linnaeus created a hierarchical system to organize living organisms by likeness, starting with the general rank of kingdom to the specific rank of species. This system is a phenetic system based on the similarity of existing features and adaptations. Additionally, he created a naming system called binomial nomenclature, or binomial naming. At the time Linnaeus created his system, botanists named ‘new’ organisms whatever they liked, making everything quite confusing. Remember, this is a time of European expansion and non-European species were being encountered relatively quickly. Linnaeus proposed using a genus and species name in Latin for each type of organism (Park 2014). While this did help organize species better, it would be quite some time before codes of nomenclature would be developed, e.g., the International Code of Zoological Nomenclature and the International Code of Nomenclature for Algae, Fungi, and Plants. While the seven taxonomic ranks created by Linnaeus are still most used, many more ranks have been developed over the years, allowing biologists to be ever more specific.
As our knowledge of evolutionary processes grew, a new approach to creating relationships developed based on evolutionary relationships. This new system is called cladistics (also called theevolutionary or phylogenetic approach). In this approach, anatomical and genetic evidence is used to construct clades, or groups of organisms that evolved from a common ancestor (Larsen 2022, 160). The resulting diagrams look like branching trees.
While all classification approaches attempt to represent reality, it is important to remember that these approaches are tools that help scientists construct a hypothesis about relationships between organisms. Using DNA helps us better reflect reality, and the more fossil data collected, the closer we can get to that reality.
Primate Classification
There are many kinds of living primates in the world today. The International Union for Conservation of Nature (IUCN) identifies over 600 species of living primates (“Primates in Peril” 2012). To identify relationships between the many species, anthropologists have used a traditional, or gradistic, approach and an evolutionary, or cladistic, approach to primate classification. The traditional approach groups primates into two suborders called Prosimians and Anthropoids using comparative anatomy. The evolutionary approach also identifies two suborders, Strepsirrhines and Haplorrhines , but uses evolutionary relationships to establish taxonomic ranks. The results of the two approaches are similar with one important difference: the traditional approach identifies tarsiers as Prosimians because of their similar physical features and the evolutionary approach places tarsiers with the haplorrhines because it is argued that tarsiers share a common ancestor with monkeys, apes, and humans, not lemurs, galagos, and lorises (Larsen 2022). This class takes an evolutionary approach to primate classification.
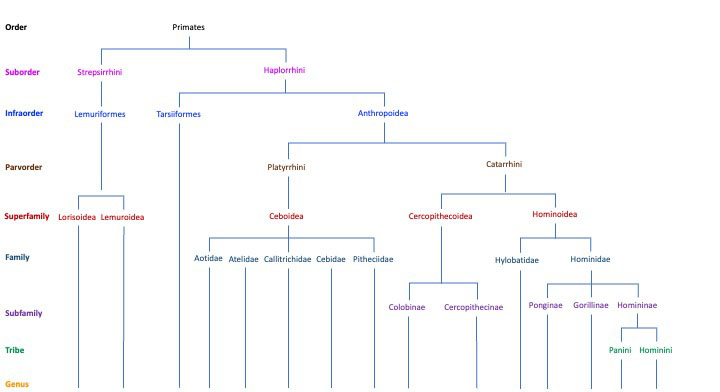
Order Primate
There are several traits that differentiate primates from other mammals. This does not mean that other mammals do not have these traits, but this combination is unique to primates and are considered ancestral or primitive traits. Ancestral traits are those characteristics that are found in multiple species of a group, including the oldest identified species of that group, as opposed to derived traits which are characteristics that are unique to only one or a few species in a group (Larsen 2022, 160). The following derived traits differentiate primates from other mammals.
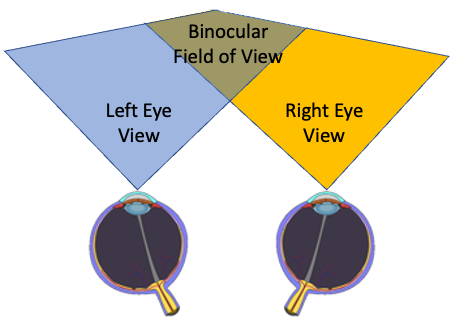
Vision is both stereoscopic and binocular. Primates have an overlapping field of vision (stereoscopic) allowing for depth perception. Binocular vision means that primates’ eyes see the same thing.
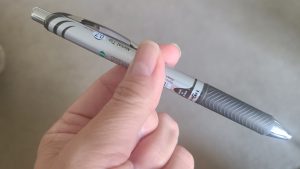
Opposable thumbs and halluces (big toes). Most primates have thumbs and halluces that can move opposite to and touch other digits of the same hand or foot that allows for grasping and manipulation of objects.
Flat nails. This ancestral trait provides protection for the ends of the fingers and toes, and makes the manipulation of objects easier.
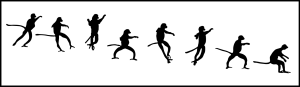
Flexible skeletal structure. Primates’ flexible skeletal structure allows for greater ease of movement. “…the bones making up the shoulders, limbs, hands, and feet tend to separate, and these separate bones are articulated at highly mobile joints” (Larsen 2022, 150).
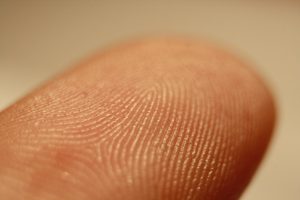
Enhanced sense of touch. The ends of primates’ fingers and toes are more sensitive than those of our mammalian relatives. The dermal ridges (prints) provide resistance to slipping and enhance the sense of touch.
Reduced olfactory sense. Primates rely less on their sense of smell than other mammals. Their snouts are shorter, which means there is less surface area inside of the nose to capture scents.
Reduced number of teeth. Primates have fewer teeth than the mammalian ancestor. The dental formula, or the number of incisors, canines, premolars, and molars in one half of the upper or lower jaw, helps to classify primates.
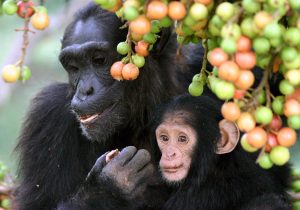
Parental investment. Primate parents invest more in childcare than other mammals because of maturation rates, which are slower because of the time needed for the development of cognitive abilities, including social learning. The length of parental investment time is variable depending on maturation rates. For instance, orangutan mothers may spend around 8 years rearing their offspring, while human parents invest the most time and energy, up to an 18-year childhood (the length of childhood is culturally determined for humans).
Social living. Most primates live in social groups, although there are exceptions such as tarsiers and orangutans. How this differs from other species that live in groups is that primates “…recognize individuals. A primate group is made up of the collective relationships among all the individuals who are members” (Park 2014, 109). Distinctive markings on faces, such as coloration, help individuals to identify one another. Primate social groups have a dominance hierarchy, which is a system of social ranking. While the dominant member in most primate species is male, in some species, e.g., ring-tail lemurs and bonobos, females hold dominant positions.
Suborder Strepsirrhini
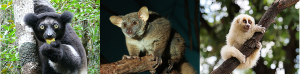
The strepsirrhines are comprised of two superfamiles: Lemuroidea which includes lemurs and aye ayes, and Lorisoidea which includes lorises, and galagoes (also known as bush babies). While there are hundreds of taxa in this suborder, there are numerous characteristics that unite the diverse species. The strepsirrhines are considered the most primitive of the primates, meaning that they retain many ancestral characteristics, and are probably more closely related to the ancestral primate. They display the most diversity among the primates. This is important as it means they have been around longer for mutations to accumulate.
The strepsirrhines include the smallest living primates, from the mouse lemur weighing in at about 1 ounce to the Indri at approximately 20 pounds. All strepsirrhines have a moist rhinarium. In other words, they have a wet nose, which allows them to capture more scents than their haplorrhine (hăp′plə-rīn′) cousins. Additionally, they have enlarged nasal passages and a large olfactory bulb in the front brain that controls their sense of smell. Some even practice territorial scent marking using urine. This means that of all the primates, strepsirrhines rely more on their non-vision senses.
Strepsirrhines exhibit diverse diets. Aye ayes and the Lorisidae are insectivores, also called faunivores, which means they rely primarily on insects for their diet. Lemurs display a range of diets from the gummivorous fork-marked lemur (Phaner furcifer) meaning it eats gums and tree saps, to the herbivorous Indri (Indri indri) and frugivorous black-and-white ruffed lemur (Varecia variegatta), which eats predominantly fruit.
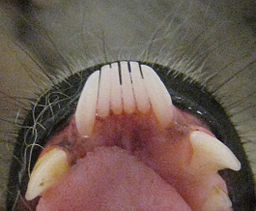
Most strepsirrhines have a tooth comb that is used for grooming and scraping up gummy foods like tree sap, and a grooming or toilet claw, which is a claw on the second toe that is used for, unsurprisingly, grooming.
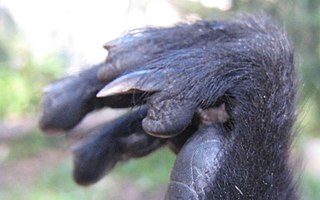
Their eyes are more laterally placed, and they see mostly in black and white. Most are nocturnal (active at night), although some of the larger lemurs display varying activity patterns, e.g., ring tail lemurs (Lemur catta) are cathemeral (active at various times during the 24-hour period), greater bamboo lemurs (Prolemur simus) are crepuscular (active at dawn and dusk), and brown lemurs (Eulemur fulvus) are diurnal (active during the day). To help them see in the dark, all strepsirrhines have a tapetum lucidum, which is a reflective area at the back of the eye. This area reflects light, allowing these primates to see better in low light conditions (Etting 2019). Strepsirrhine’s eyes do not have a postorbital closure, meaning the eye sockets are not enclosed. Instead, they have a postorbital bar.
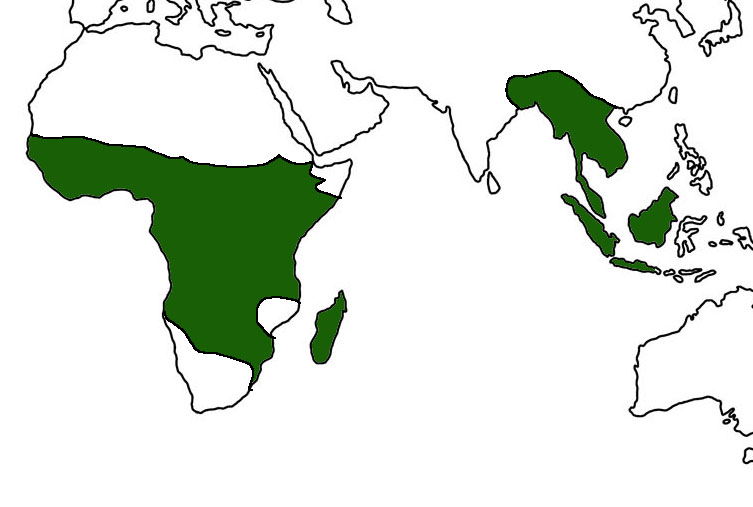
Strepsirrhines have a relatively restricted distribution. They are found in sub-Saharan Africa, Madagascar and the Comoro Islands, along with parts of southeast Asia, Singapore, and Malaysia.
This is by no means an exhaustive overview of the strepsirrhines. You can learn more about this suborder at Meet the Living Primates.
Suborder Haplorrhini
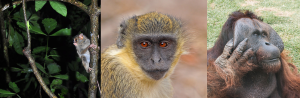
The haplorrhines are divided into two infraorders: Tarsiiformes and Anthropoidea. Tarsiiformes are comprised of only one genus, Tarsius. The anthropoids consist of monkeys and apes, including humans, with hundreds of different species. Similarly to the strepsirrhines, there are some general derived characteristics that unite the haplorrhines.
Unlike the strepsirrhines, haplorrhines have a dry rhinarium and shorter snouts, resulting in an increased reliance on vision. They lack the tapetum lucidum so that almost all haplorrhines are diurnal or active during the day. Owl monkeys (genus Aotus) are a notable exception; however, they have derived traits such as large eyeballs and more rods than cones in the retina (rods and cones are photoreceptors; rods work better in low light and cones are used to see color). Eyes are fully rotated forward, and all haplorrhines see in color, albeit the owl monkeys do not see color as well as other haplorrhines. The eye orbit is enclosed—the postorbital closure mentioned previously—which helps to protect the eyes. Haplorrhines can also see up close in detail unlike the strepsirrhines.
Haplorrhines have larger brains than their strepsirrhine cousins. They are bigger in general. The smallest haplorrhines are the tarsiers and pygmy marmosets, both weighing approximately 5 ounces. The largest haplorrhine is the gorilla. Male gorillas can weigh close to 500 pounds, which is about twice as much as female gorillas. This difference in weight between the two sexes is a form of sexual dimorphism, which are differences in morphological characteristics between the sexes. Sexual dimorphism is common among the many haplorrhine species, particularly as it relates to size.
Because haplorrhines are generally larger than strepsirrhines, they cannot rely on insects for their diet. Tarsiers are faunivores (carnivores), relying on insects, birds, lizards, and other small animals. The anthropoids display a wide variety of diet from frugivores (fruit), folivores (leaves), and herbivores (mixed plants), to omnivores (a bit of everything).
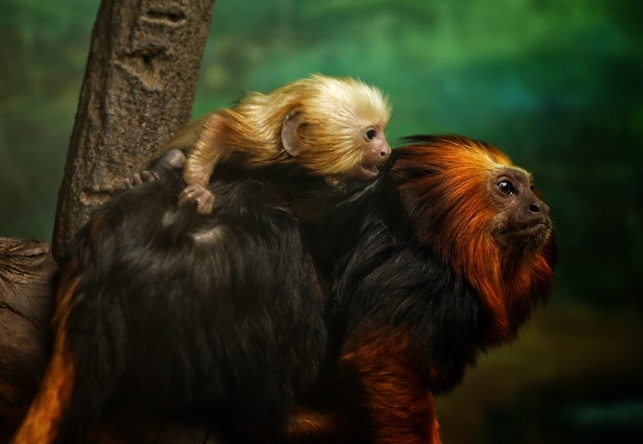
Behaviorally, there is more mutual grooming among the haplorrhines and there are longer gestation and maturation periods resulting in increased parental investment. There are some primate species where male parental investment equals that of the female. In fact, among Leontopithecus rosalia, or the golden lion tamarin, males are responsible for most of the infant care. Mothers only take the infants for feeding.
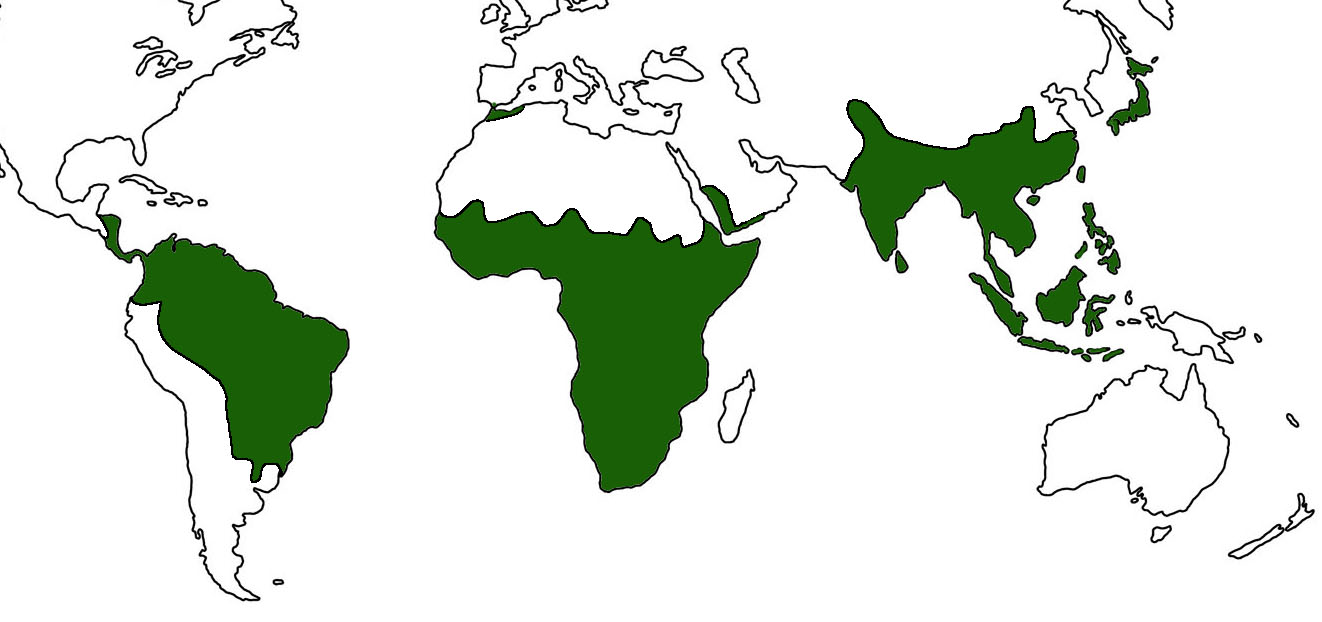
Haplorrhines have a wide geographic distribution. Excluding humans, the haplorrhines inhabit most areas of the southern hemisphere. The northern-most species, Japanese macaques (Macaca fuscata) are found on all the Japanese islands, except Hakkaido (Gron 2007). An examination of the map above clearly shows some areas of the southern hemisphere lacking primates.
In South America, primates are not naturally found west of the Andean Mountains, suggesting the mountain range prohibited the distribution of primate species. Australia and other Oceanic islands also lack haplorrhines (and strepsirrhines for that matter). Using data from the earth sciences and zoology, researchers concluded that these islands split from the mainland of southeast Asia, causing reproductive isolation among animals and plants. This demarcation, first noted by Alfred Russel Wallace in the 19th century, is called the Wallace Line. West of the Wallace line, species are associated with the Asian mainland. East of the line, species are similar to or derived from Australian species (Scoville 2020).
You can learn more about this suborder at Meet the Living Primates.
Primate Evolution
What anthropologists know about primate evolution comes from the fossilized remains of animals and plants. Why plants? Knowing about the paleoenvironment helps us to better understand the behaviors that fossil species might have displayed to survive and reproduce. In fact, angiosperms, or flowering plants, appear in the fossil record about the same time as primates, leading some to hypothesize that they co-evolved. The angiosperm hypothesis states that a mutually beneficial relationship evolved between angiosperms and primates. New resources were available to animals at the terminal ends of branches and the animals dispersed the seeds of the angiosperms (Sussman 1991). The angiosperm hypothesis is one of three primary hypotheses about the origins of primates. The first hypothesis proposed that the suite of primate characteristics evolved in response to life in the trees (arboreal hypothesis), while the visual predation hypothesis suggests that primate characteristics evolved because they were an advantage for hunting small insects. You can read about a recent controversial hypothesis explaining the role of volcanic eruptions in primate evolution at Live Science.
Fossils
Fossils, the remains of living organisms, are formed through several processes (Castro 2015):
- Compression: the dark imprint of organism caused by compression at high pressure. This process is also called carbonization or distillation.
- Molds and casts: the impression of organism made in sediment leaving a depression, which is then filled with other sediment to create a cast of the organism.
- Permineralization: the most common type of fossilization process occurs when organic material is buried in sediment. It decays and is replaced by minerals, e.g., silica, brought in by water.
- Preserved remains: in rare instances, organic material is frozen, desiccated, or encased in something like amber. This rare process preserves organic remains as close to the living organism as possible.
- Replacement: sometimes organic material such as shell or bone dissolves and is replaced by a different mineral, e.g., when a calcite shell is replaced by quartz. If permineralization also occurs then the organism has petrified, which is the process of turning organic material into stone.
It is important to acknowledge that our knowledge of the fossil record is incomplete for several reasons: many fossils are not yet discovered and many that have been found are waiting to be described. Also, the fossilization processes themselves bias the fossil record in that organisms must have died in the right conditions to be fossilized and harder organic material fossilizes better than softer organic material. Additionally, the geological record is incomplete as erosion eats away at earth’s surface, hence, not all geologic levels are found everywhere. However, “…even though no complete sequence of rocks exists anywhere on Earth, nearly all intervals of geological time are present somewhere, and often in multiple places…allowing a generalized understanding of Earth’s long history to be determined” (Hendricks 2019). This is not unlike historical records, which are also incomplete and contain biases.
Geologic Time Scale
The geologic time scale is the earth science’s equivalent to chemistry’s periodic table (Hendricks 2018) and biology’s binomial nomenclature. It is a communication system that allows scientists to communicate effectively. In referencing one of the scale’s categories of time, one scientist should be able to know exactly what another scientist is talking about without having details. For instance, when we read that a hominid fossil was found dating to the Miocene, our mind should correlate that to 25.5 to 5.3 million years ago (mya).
The geologic time scale is divided into five component parts, from the broadest (eons) to the smallest (ages):
- Eons
- Eras
- Periods
- Epochs
- Ages
For the purposes of this class, we are not going to look at Ages, so they are left off the following table. You should make yourself familiar with the components of the Cenozoic Era.
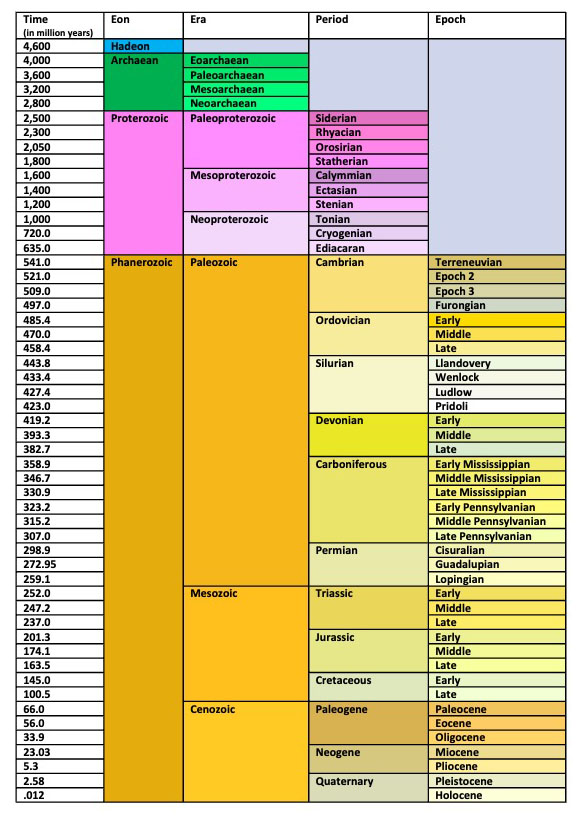
In recent years, a debate has started on whether to rename the Holocene to the Anthropocene to better illustrate the impact humans have had on earth’s ecosystems, or whether to leave the geologic time scale in its current state. As of the writing of this chapter, the International Union of Geological Sciences, the organization in charge of defining the geologic time scale, had not yet decided.
Anthropological interest starts in the Cenozoic Era, when the first proto-primates appear in the fossil record. We can refer to this time frame as the adaptive radiation of the primates. Adaptive radiation occurs when a group of organisms diversifies into new forms adapted for specific environments (Larsen 2022, G11).
Adaptive Radiation of the Primates
In this section, we will briefly explore the evolution of primates, starting with the mammalian ancestor of all living primates.
Mammals first appear in the fossil record about 300 million years ago towards the end of the Carboniferous period shortly (geologically speaking) before the mass extinction event caused by an asteroid hitting earth. This extinction event, known as the Cretaceous-Paleogene Boundary (K-Pg boundary) wiped out dinosaurs, providing an opportunity for the adaptive radiation of mammals. Computer models developed by Hughes et.al. (2021) suggest that while most of the surviving mammalian species were non-arboreal, the ancestors of primates and marsupials were arboreal, filling new ecological niches opened after the extinction event.
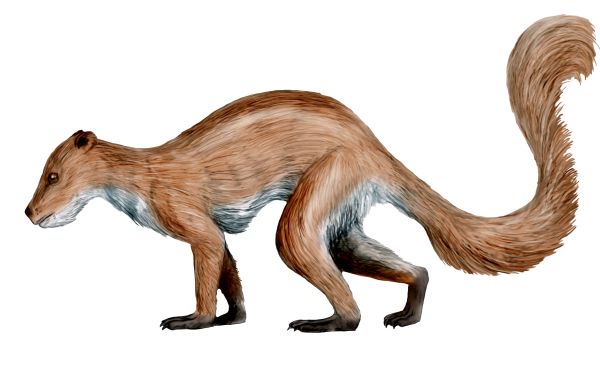
Based on current data but still debated, the mammal most likely ancestral to primates is Plesiadapis. These proto-primates appear in the fossil record during the Paleocene epoch (66-56 mya) and no longer appear by the beginning of the Eocene. They were small arboreal mammals, similar in size to modern squirrels and tree shrews. The oldest plesiadapiform fossils are from North America, suggesting that the order Primate originated there (Larsen 2022); however, plesiadap fossils are found in Europe and Asia as well, further suggesting they migrated across land bridges.
As mentioned above, it is debated among paleontologists whether Plesiadapis is the ancestor of primates. This is because the paleospecies does not display all of the characteristics associated with primates. However, this should not be surprising as it is highly unlikely that the key characteristics evolved all at the same time. The over 140 Plesiadapis species do share some traits such as grasping fingers and divergent halluces with nails. Additionally, they have some similar dental characteristics, e.g., similar cusps and crests (Silcox 2014). Nonetheless, researchers agree that the closest living mammalian relative is the treeshrew (Scandentia). This species is often used as a model for the first species in the order Primate. “Indeed, the most primitive plesiadapiform known from a reasonably complete skeleton…resembles the pen-tailed treeshrew, Ptilocercus lowii…these similarities suggest that the first primates probably looked quite a bit like Ptilocercus, and supports the idea that primates evolved from an already arboreal ancestor” (Silcox 2014, 1).
The first true primates, i.e., euprimates, emerge during the Eocene (about 56-34 mya). Resembling modern strepsirrhines, the euprimates possess key primate traits such as grasping hands and feet, shorter snouts, nails, and forward-facing eyes. They also had larger brains in relation to body size, another hallmark of primates. There are two types of euprimates: adapids and omomyids. Adapids are called ‘stem strepsirrhines’ because they most closely resemble modern strepsirrhines such as lemurs. Omomyids are ‘stem haplorrhines’ and appear tarsier-like with a shorter skull and large eye orbits. By 55 mya, we see the first characteristics of haplorrhines appear in Archicebus, a fossil species from China, which has some features of tarsiers and monkeys. The skull is tarsier-like with a short snout, small eye orbits, and high, pointed molar cusps. Its monkey-like traits include heel and ankle bones. Based on its characteristics, researchers suggest that Archicebus was a small (~ 1 ounce), diurnal, arboreal, insectivorous primate (Larsen 2022). By the middle Eocene, ~42 mya, primates with anthropoid traits appear in the fossil record. In fact, the genus name, Eosimias, translates to ‘dawn monkey.’ It is considered the first true anthropoid as it shares some characteristics with modern anthropoids, such as its ankle and jaw structure. Recently, the oldest ancestor of modern monkeys in South America was described. Perupithecus looks more like Eocene African anthropoids than other New World paleospecies or modern platyrrhines, suggesting an African origin for New World monkeys (Bond et.al. 2015). While we cannot say definitively how primates arrived in South America, there are two likely scenarios: 1) they rafted on mats of vegetation (the continents were closer together in the Eocene and Oligocene than they are today) or 2) they island hopped across the “historically much warmer South Atlantic and Antarctic regions” (Klaus 2011, 301).
The adaptive radiation of the anthropoids occurred during the Oligocene (roughly 34-23 mya) as a globally cooling climate opened new ecological niches (Klaus 2011). The various species share numerous traits, including increased reliance on vision, shortened snouts, larger brains, fully enclosed eye orbits, and convergent eyes. Most of what is known about Oligocene anthropoids comes from the Fayyum Depression in Egypt where the ancestors of both strepsirrhines and haplorrhines have been found. The oldest of the anthropoids are the oligopithecids, dating to ~35 mya, one of three groups of anthropoids found in the Fayyum. Bond et.al. (2015) propose that it is the extinct oligopithecid, Talahpithecus, that is the ancestor of Perupithecus. Propliopithecids and parapithecids are the other two groups of anthropoids from the Fayyum. Aegyptopithecus, a propliopithecid dating to 32-29 mya, is most likely ancestral to all catarrhines, or Old World monkeys. These anthropoid groups were highly successful as indicated by fossils found not only in Africa, but Pakistan, China, and Mongolia (Larsen 2022).
In the late Oligocene, we have evidence of the split between Old World monkeys and apes between 30 to 25 mya. Fossil data from the Rukwa Rift Basin, Tanzania, clearly demonstrates that Old World monkeys and ancestral apes were both present by 25 mya. Found was Rukwapithecus fleaglei, a hominoid as indicated by dentition, and Nsungwepithecus gunnelli, a cercopithecoid also as indicated by dentition (Larsen 2022).
The Miocene ushers in the adaptive radiation of apes (over 50 hominoid genera) as a warming trend once again causes changes to the environment, particularly the expansion of the tropical rain forest. While there are several derived traits separating apes from monkeys, the Y-5 molar pattern is one of the most indicative, particularly since teeth are the hardest substance in the body and the most likely to preserve. Many of the post-cranial traits we now associate with apes evolved after changes in dentition. Micropithecus and Proconsul are two of the earliest apes to appear in the Miocene fossil record. The fossils of these early apes are found across a wide range of habitats and their teeth indicate that there was a variety of diets employed, although all seem to have enjoyed ripe fruit. Proconsul’s foot and wrist bones suggest that it was arboreal. Morotopithecus is one of the oldest apes found in Africa. Dated to ~20.6 mya, its skeleton suggests that it was capable of modern ape behavior.
Fossil apes have been found in Europe and Asia as well as Africa by about 17 mya. The movement of these paleospecies of apes was facilitated by lower sea levels that created land bridges across the Tethys Sea. Dryopith apes like Dryopithecus found in the European fossil record from 15-7 mya “…consistently exhibit postcranial features of living hominoids” (Almécija et.al. 2021). It has been proposed that one of the dryopiths, Danuvius, exhibits adaptations to habitual bipedalism (Böhme et al. 2019) although this is a debated topic (Williams et.al. 2020). Apes found in Asia and Indonesia indicate a broad distribution of ancestral hominoids. Khoratpithecus (9-6 mya), found in Thailand and Myanmar, may be ancestral to modern orangutans (Pongo). Sivapithecus is the oldest Asian ape found to date. At 12-8mya, it was adapted for eating hard foods like seeds and nuts. One of the most interesting of these early ape species is Gigantopithecus, a paleospecies related to orangutans. As the genus name implies, this was an extremely large ape. Fossils recovered from China, India, and Vietnam inform us that it was about 10 feet tall and may have weighed up to 660 pounds. Gigantopithecus first appears in the fossil record about 8 mya and disappears by 0.3 mya (Larsen 2022).
About 8 mya, the climate started to warm. The Mediterranean Sea dried up and savannas and grasslands began to spread in eastern Africa. Ape species begin to dwindle until today we are left with only five ape genera: Hylobates (gibbons and siamangs), Pongo (orangutans), Gorilla, Pan (chimpanzees and bonobos), and Homo (humans). During this late Miocene period, Hlusko et.al. (2016) suggest that monkeys were able to proliferate, taking over niches once inhabited by apes either because of dietary causes or the life cycle. It is also sometime during this time frame of the late Miocene that the common ancestor of humans and chimpanzees lived.
References
Böhme M., N. Spassov, J. Fuss, A. Tröscher, A. S. Deane, J. Prieto, U. Kirscher, T. Lechner, and D. R. Begun. 2019. “A New Miocene Ape and Locomotion in the Ancestor of Great Apes and Humans.” Nature 575, 489–493 (2019). Accessed March 22, 2022. d.o.i. 10.1038/s41586-019-1731-0.
Bond, Mariano, Marcelo F. Tejedor, Kenneth E. Campbell, Jr., Laura Chornogubsky, Nelson Novo, and Francisco Goin. 2015. “Eocene Primates of South America and the Africa Origin of New World Monkeys.” Nature 520: 538-541. Accessed March 22, 2022. https://doi-org.offcampus.lib.washington.edu/10.1038/nature14120.
Castro, Joseph. 2015. “How Do Fossils Form?” LiveScience. Accessed March 21, 2022. https://www.livescience.com/37781-how-do-fossils-form-rocks.html.
Biological Anthropology, edited by Beth Shook, Katie Nelson, Kelsie Aguilera, and Lara Braff. Arlington, VA: American Anthropological Association. Accessed March 17, 2022. https://pressbooks-dev.oer.hawaii.edu/explorationsbioanth/chapter/__unknown__-4/.
Helmenstine, Anne Marie. 2019. “Golden Lion Tamarin Facts.” ThoughtCo. Accessed March 21, 2022. https://www.thoughtco.com/golden-lion-tamarin-facts-4583938.
Hendricks, Jonathan R. 2018. “Geological Time.” In Digital Encyclopedia of Ancient Life. Ithaca, NY: Paleontological Research Institution. Accessed March 21, 2022. https://www.digitalatlasofancientlife.org/learn/geological-time/.
Hendricks, Jonathan R. 2019. “Nature of the Fossil Record.” In Digital Encyclopedia of Ancient Life. Ithaca, NY: Paleontological Research Institution. Accessed March 21, 2022. https://www.digitalatlasofancientlife.org/learn/nature-fossil-record/completeness-of-the-fossil-record/.
Hlusko, Leslea J., Christopher A. Schmitt, Tesla A. Monson, Marianne F. Brasil, and Michael C. Mahaney. 2016. “The Integration of Quantitative Genetics, Paleontology, and Neontology Reveals Genetic Underpinnings of Primate Dental Evolution.” Proceedings of the National Academy of Sciences 113(33). Accessed March 22, 2022. https://www.pnas.org/doi/full/10.1073/pnas.1605901113.
Howard Hughes Medical Institute. 2005. “Evidence That Human Brain Evolution Was a Special Event.” ScienceDaily. Accessed March 28, 2022. https://www.sciencedaily.com/releases/2005/01/050111165229.htm.
Hughes, Jonathan J., Jacob S. Berv, Stephen G. B. Chester, Eric J. Sargis, and Daniel J. Field. 2021. “Ecological Selectivity and the Evolution of Mammalian Substrate Preference Across the K-Pg Boundary. Ecology and Evolution October 11. Accessed March 22, 2022. DOI: 10.1002/ece3.8114.
Klaus, Haagen D. 2015. “Non-Human Primate and Human Evolution.” In Grizmek’s Animal Life Encyclopedia: Evolution, edited by Michael Hutchins, 299-309. Detroit, MI: Gale. Accessed March 22, 2022. https://link.gale.com/apps/doc/CX1919700042/GVRL?u=wash_main&sid=bookmark-GVRL&xid=a4131da5.
LaFleur, Marni, Michelle Sauther, Frank Cuozzo, Nayuta Yamashita, Ibrahim Antho Jacky Youssouf, and Richard Bender. 2014. “Cathemerality in Wild Ring-Tailed Lemurs (Lemur catta) in the Spiny Forest of Tsimanampetsotsa National Park: Camera Trap Data and Preliminary Behavioral Observations.” Primates 55(2): 207-217. Accessed March 17, 2022. doi:10.1007/s10329-013-0391-1.
Larsen, Clark Spencer. 2022. Essentials of Biological Anthropology, 5th edition. New York, NY: W. W. Norton & Company, Inc.
Lewis, Ricki. 2016. “Taxonomy, History of.” In Biology, 2nd ed., edited by Melissa Sue Hill, 157-160. Vol. 4. Farmington Hills, MI: Macmillan Reference USA. Accessed March 16, 2022. https://link.gale.com/apps/doc/CX3629800414/GVRL?u=wash_main&sid=bookmark-GVRL&xid=1fcf03b9.
Malmquist, Sarah and Kristina Prescott. n.d. Human Biology. Accessed March 22, 2022. https://open.lib.umn.edu/humanbiology/chapter/1-7-the-evolution-of-primates/.
Park, Michael Alan. 2014. Introducing Anthropology: An Integrated Approach, 6th edition. New York, NY: McGraw-Hill Education.
“Primates in Peril – Conservationists Reveal the World’s 25 Most Endangered Primates.” International Union for Conservation of Nature. Accessed March 16, 2022. https://www.iucn.org/content/primates-peril-conservationists-reveal-worlds-25-most-endangered-primates.
Robinson, Richard. 2016. “Linnaeus, Carolus.” In Biology, 2nd ed., edited by Melissa Sue Hill. Vol. 3. Farmington Hill, MI: Macmillan Reference USA. Accessed March 16, 2022. https://link.gale.com/apps/doc/CX3629800256/GVRL?u=wash_main&sid=bookmark-GVRL&xid=f1a3d5f8.
“Rods and Cones of the Human Eye.” n.d. Ask a Biologist, Arizona State University. Accessed March 17, 2022. https://askabiologist.asu.edu/rods-and-cones.
Saneda, Tori and Michelle Field. 2015. “Classification.” Accessed March 16, 2022. https://wikieducator.org/Biological_Anthropology/Classification.
Saneda, Tori and Michelle Field. 2015. “Primate Evolution.” Accessed March 21, 2022. https://wikieducator.org/Biological_Anthropology/Unit_2:_Non-human_Primates/Primate_Evolution.
Scoville, Heather. 2020. “What Is the Wallace Line?” ThoughtCo. Accessed March 21, 2022. https://www.thoughtco.com/the-wallace-line-1224711.
Silcox, Mary T. 2014. “Primate Origins and the Plesiadapiforms.” Nature Education Knowledge 5(3): 1. Accessed March 22, 2022. https://www.nature.com/scitable/knowledge/library/primate-origins-and-the-plesiadapiforms-106236783/.
Szymik, Brett. 2011. “What’s in Your Brain?” ASU-Ask a Biologist. Accessed March 28, 2022. https://askabiologist.asu.edu/parts-of-the-brain.
Sussman, Robert W. 1991. “Primate Origins and the Evolution of Angiosperms.” American Journal of Primatology 23(4): 209-223. Accessed March 21, 2022. doi: 10.1002/ajp.1350230402.
University of Colorado Denver. 2012. “Evolutionary Increase in Size of the Human Brain Explained: Part of a Protein Linked to Rapid Change in Cognitive Ability.” ScienceDaily. Accessed March 28, 2022. https://www.sciencedaily.com/releases/2012/08/120816141537.htm.
Walker, J. D., J. W. Geissman, S. A. Bowring, and L. E. Babcock. 2018. “Geologic Time Scale v. 5.0: Geological Society of America. Accessed March 21, 2022. https://www.geosociety.org/documents/gsa/timescale/timescl.pdf.
Whitaker, Roger M. Gualtiero B. Colombo, Stuart M. Allen, and Robin I. M. Dunbar. 2016. “A Dominant Social Comparison Hueristic United Alternative Mechanisms for the Evolution of Indirect Reciprocity.” Scientific Reports 6: 31459. Accessed March 28, 2022. https://doi.org/10.1038/srep31459.