5.5-Mechanisms of Other Antimicrobial Drugs
10.3 Mechanisms of Other Antimicrobial Drugs
Learning Objective
- Explain the differences between modes of action of drugs that target fungi, protozoa, helminths, and viruses
Because fungi, protozoa, and helminths are eukaryotic, their cells are very similar to human cells, making it more difficult to develop drugs with selective toxicity. Additionally, viruses replicate within human host cells, making it difficult to develop drugs that are selectively toxic to viruses or virus-infected cells. Despite these challenges, there are antimicrobial drugs that target fungi, protozoa, helminths, and viruses, and some even target more than one type of microbe. Table 10.7, Table 10.8, Table 10.9, and Table 10.10 provide examples for antimicrobial drugs in these various classes.
Antifungal Drugs
The most common mode of action for antifungal drugs is the disruption of the cell membrane. Antifungals take advantage of small differences between fungi and humans in the biochemical pathways that synthesize sterols. The sterols are important in maintaining proper membrane fluidity and, hence, proper function of the cell membrane. For most fungi, the predominant membrane sterol is ergosterol. Because human cell membranes use cholesterol, instead of ergosterol, antifungal drugs that target ergosterol synthesis are selectively toxic (Figure 10.7).
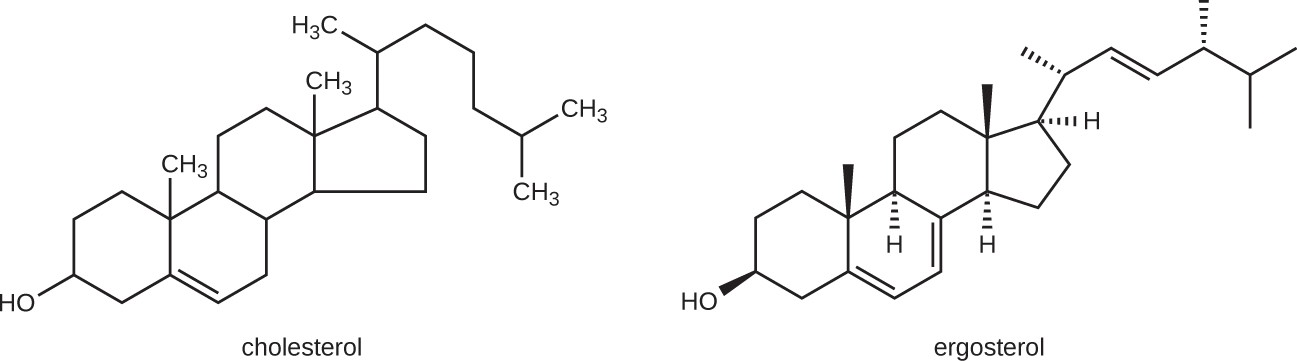
Beyond targeting ergosterol in fungal cell membranes, there are a few antifungal drugs that target specific substances found in fungal cell walls, interfere with fungal cell division, or act as antimetbolites against fungal processes.
Table 10.7 shows the various therapeutic classes of antifungal drugs, categorized by mode of action, with examples of each.
Mechanism of Action |
Drug Class |
Specific Drugs |
Clinical Uses |
Inhibit ergosterol synthesis |
Imidazoles |
Miconazole, ketoconazole, clotrimazole |
Fungal skin infections and vaginal yeast infections |
Triazoles |
Fluconazole |
Systemic yeast infections, oral thrush, and cryptococcal meningitis |
|
Allylamines |
Terbinafine |
Dermatophytic skin infections (athlete’s foot, ring worm, jock itch), and infections of fingernails and toenails |
|
Bind ergosterol in the cell membrane and create pores that disrupt the membrane |
Polyenes |
Nystatin |
Used topically for yeast infections of skin, mouth, and vagina; also used for fungal infections of the intestine |
Amphotericin B |
Variety systemic fungal infections |
||
Inhibit cell wall synthesis |
Echinocandins |
Caspofungin |
Aspergillosis and systemic yeast infections |
Not applicable |
Nikkomycin Z |
Coccidioidomycosis (Valley fever) and yeast infections |
|
Inhibit microtubules and cell division |
Not applicable |
Griseofulvin |
Dermatophytic skin infections |
Table 10.7

- How is disruption of ergosterol biosynthesis an effective mode of action for antifungals?
Antiprotozoan Drugs
There are a few mechanisms by which antiprotozoan drugs target infectious protozoans (Table 10.8). Some are antimetabolites, others interfere with nucleic acid synthesis, and one class interferes with heme detoxification, which is necessary for the parasite’s effective breakdown of hemoglobin into amino acids inside red blood cells.
Mechanism of Action |
Drug Class |
Specific Drugs |
Clinical Uses |
Inhibit electron transport in mitochondria |
Naphthoquinone |
Atovaquone |
Malaria, babesiosis, and toxoplasmosis |
Inhibit folic acid synthesis |
Not applicable |
Proquanil |
Combination therapy with atovaquone for malaria treatment and prevention |
Sulfonamide |
Sulfadiazine |
Malaria and toxoplasmosis |
|
Not applicable |
Pyrimethamine |
Combination therapy with sulfadoxine (sulfa drug) for malaria |
|
Produces damaging reactive oxygen species |
Not applicable |
Artemisinin |
Combination therapy to treat malaria |
Inhibit DNA synthesis |
Nitroimidazoles |
Metronidazole, tinidazole |
Infections caused by Giardia lamblia, Entamoeba histolytica, and Trichomonas vaginalis |
Not applicable |
Pentamidine |
African sleeping sickness and leishmaniasis |
|
Inhibit heme detoxification |
Quinolines |
Chloroquine |
Malaria and infections with E. histolytica |
Mepacrine, mefloquine |
Malaria |
Table 10.8

- List two modes of action for antiprotozoan drugs.
Antihelminthic Drugs
Because helminths are multicellular eukaryotes like humans, developing drugs with selective toxicity against them is extremely challenging. Despite this, several effective classes have been developed (Table 10.9). Some bind to helminthic β-tubulin, preventing microtubule formation. Microtubules in the intestinal cells of the worms seem to be particularly affected, leading to a reduction in glucose uptake. Another type of drug binds to glutamate-gated chloride channels specific to invertebrates including helminths, blocking neuronal transmission and causing starvation, paralysis, and death of the worms. Many antihelminthic drugs have modes of action that are unclear, but appear to interfere with ATP formation, inhibit RNA synthesis, or result in calcium influx into the worm.
Mechanism of Action |
Drug Class |
Specific Drugs |
Clinical Uses |
Inhibit microtubule formation, reducing glucose uptake |
Benzimidazoles |
Mebendazole, albendazole |
Variety of helminth infections |
Block neuronal transmission, causing paralysis and starvation |
Avermectins |
Ivermectin |
Roundworm diseases, including river blindness and strongyloidiasis, and treatment of parasitic insects |
Inhibit ATP production |
Not applicable |
Niclosamide |
Intestinal tapeworm infections |
Induce calcium influx |
Not applicable |
Praziquantel |
Schistosomiasis (blood flukes) |
Inhibit RNA synthesis |
Thioxanthenones |
Lucanthone, hycanthone, oxamniquine |
Schistosomiasis (blood flukes) |
Table 10.9
- Why are antihelminthic drugs difficult to develop?
Antiviral Drugs
Unlike the complex structure of fungi, protozoa, and helminths, viral structure is simple, consisting of nucleic acid, a protein coat, viral enzymes, and, sometimes, a lipid envelope. Furthermore, viruses are obligate intracellular pathogens that use the host’s cellular machinery to replicate. These characteristics make it difficult to develop drugs with selective toxicity against viruses.
Many antiviral drugs are nucleoside analogs and function by inhibiting nucleic acid biosynthesis. The mode of action of other antivirals is not entirely clear, but it appears that some prevent viral escape from the cell endosome, preventing viral replication, while others block uncoating of the viral particles inside the host cells. Neuraminidase inhibitors (i.e. Tamiflu) specifically target influenza viruses by blocking the activity of influenza virus neuraminidase, preventing the release of the virus from infected cells. These antivirals can decrease flu symptoms and shorten the duration of illness.
Viruses with complex life cycles, such as HIV, can be more difficult to treat. First, HIV targets CD4-positive white blood cells, which are necessary for a normal immune response to infection. Second, HIV is a retrovirus, meaning that it converts its RNA genome into a DNA copy that integrates into the host cell’s genome, thus hiding within host cell DNA. Third, the HIV reverse transcriptase lacks proofreading activity and introduces mutations that allow for rapid development of antiviral drug resistance. To help prevent the emergence of resistance, a combination of specific synthetic antiviral drugs is typically used to treat HIV with antiretroviral therapy (ART), including a reverse transcriptase inhibitor, a protease inhibitor, and an integrase inhibitor. (Figure 10.8).
Table 10.10 shows the various therapeutic classes of antiviral drugs, categorized by mode of action, with examples of each.
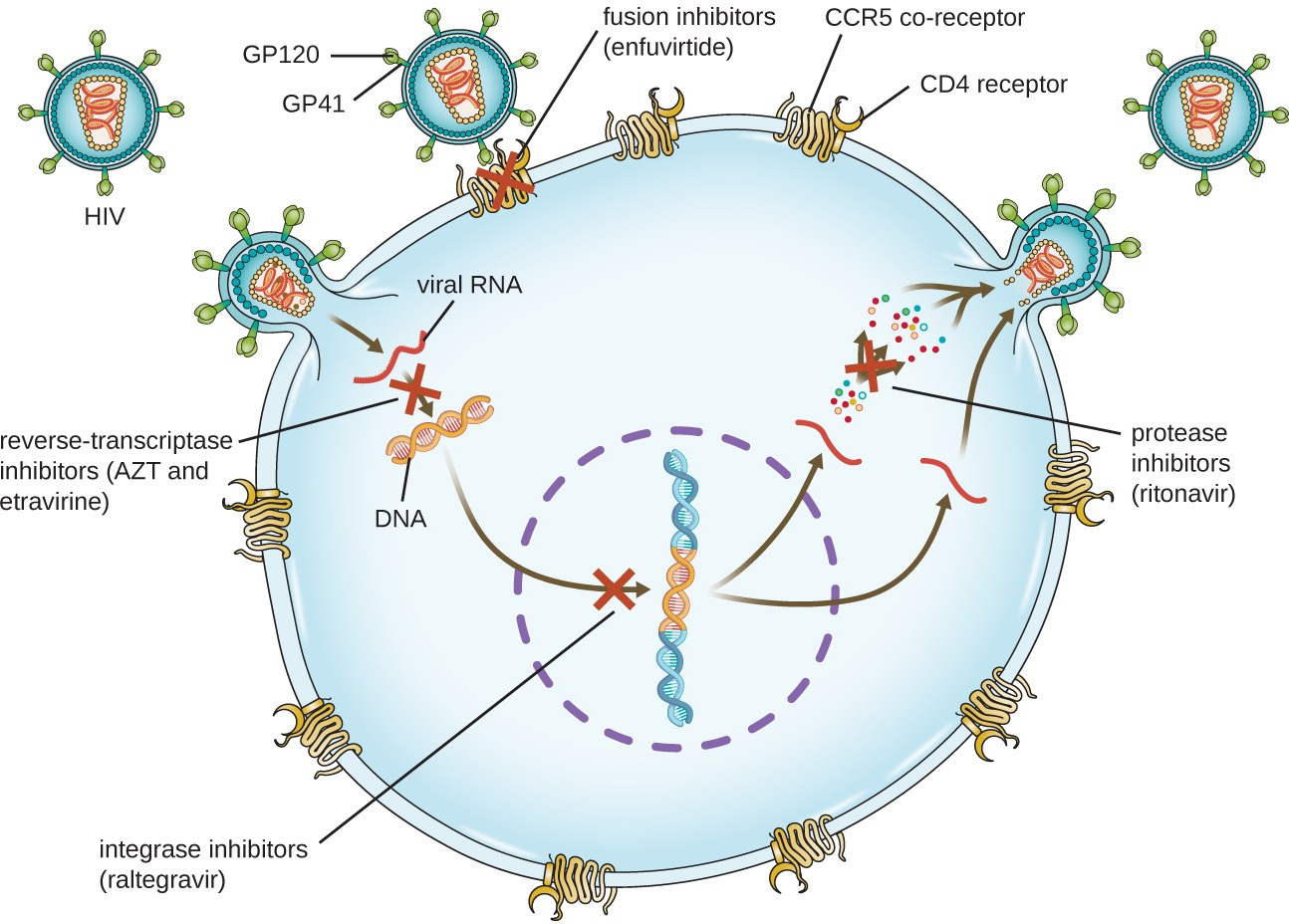
Mechanism of Action |
Drug |
Clinical Uses |
Nucleoside analog inhibition of nucleic acid synthesis |
Acyclovir |
Herpes virus infections |
Azidothymidine/ zidovudine (AZT) |
HIV infections |
|
Ribavirin |
Hepatitis C virus and respiratory syncytial virus infections |
|
Vidarabine |
Herpes virus infections |
|
Sofosbuvir |
Hepatitis C virus infections |
|
Non-nucleoside noncompetitive inhibition |
Etravirine |
HIV infections |
Inhibit escape of virus from endosomes |
Amantadine, rimantadine |
Infections with influenza virus |
Inhibit neuraminadase |
Olsetamivir, zanamivir, peramivir |
Infections with influenza virus |
Table 10.10
Mechanism of Action |
Drug |
Clinical Uses |
Inhibit viral uncoating |
Pleconaril |
Serious enterovirus infections |
Inhibition of protease |
Ritonavir |
HIV infections |
Simeprevir |
Hepatitis C virus infections |
|
Inhibition of integrase |
Raltegravir |
HIV infections |
Inhibition of membrane fusion |
Enfuviritide |
HIV infections |
Table 10.10
- Why is HIV difficult to treat with antivirals?
Link to Learning
To learn more about the various classes of antiretroviral drugs used in the ART of HIV infection, explore each of the drugs in the HIV drug classes provided by US Department of Health and Human Services at this (https://openstax.org/l/22HIVUSDepthea) website.