13 Biogeography
Learning Objectives
By the end of this section, you will be able to:
- Define biogeography
- List and describe abiotic factors that affect the global distribution of plant and animal species
- Compare the impact of abiotic forces on aquatic and terrestrial environments
- Summarize the affect of abiotic factors on net primary productivity
Many forces influence the communities of living organisms present in different parts of the biosphere (all of the parts of Earth inhabited by life). The biosphere extends into the atmosphere (several kilometers above Earth) and into the depths of the oceans. Despite its apparent vastness to an individual human, the biosphere occupies only a minute space when compared to the known universe. Many abiotic forces influence where life can exist and the types of organisms found in different parts of the biosphere. The abiotic factors influence the distribution of biomes: large areas of land with similar climate, flora, and fauna.
Biogeography
Biogeography is the study of the geographic distribution of living things and the abiotic factors that affect their distribution. Abiotic factors such as temperature and rainfall vary based mainly on latitude and elevation. As these abiotic factors change, the composition of plant and animal communities also changes. For example, if you were to begin a journey at the equator and walk north, you would notice gradual changes in plant communities. At the beginning of your journey, you would see tropical wet forests with broad-leaved evergreen trees, which are characteristic of plant communities found near the equator. As you continued to travel north, you would see these broad-leaved evergreen plants eventually give rise to seasonally dry forests with scattered trees. You would also begin to notice changes in temperature and moisture. At about 30 degrees north, these forests would give way to deserts, which are characterized by low precipitation.
Moving farther north, you would see that deserts are replaced by grasslands or prairies. Eventually, grasslands are replaced by deciduous temperate forests. These deciduous forests give way to the boreal forests found in the subarctic, the area south of the Arctic Circle. Finally, you would reach the Arctic tundra, which is found at the most northern latitudes. This trek north reveals gradual changes in both climate and the types of organisms that have adapted to environmental factors associated with ecosystems found at different latitudes. However, different ecosystems exist at the same latitude due in part to abiotic factors such as jet streams, the Gulf Stream, and ocean currents. If you were to hike up a mountain, the changes you would see in the vegetation would parallel those as you move to higher latitudes.
Ecologists who study biogeography examine patterns of species distribution. No species exists everywhere; for example, the Venus flytrap is endemic to a small area in North and South Carolina. An endemic species is one which is naturally found only in a specific geographic area that is usually restricted in size. Other species are generalists: species which live in a wide variety of geographic areas; the raccoon, for example, is native to most of North and Central America.
Species distribution patterns are based on biotic and abiotic factors and their influences during the very long periods of time required for species evolution; therefore, early studies of biogeography were closely linked to the emergence of evolutionary thinking in the eighteenth century. Some of the most distinctive assemblages of plants and animals occur in regions that have been physically separated for millions of years by geographic barriers. Biologists estimate that Australia, for example, has between 600,000 and 700,000 species of plants and animals. Approximately 3/4 of living plant and mammal species are endemic species found solely in Australia.
Australia is home to many endemic species. The (a) wallaby (Wallabia bicolor), a medium-sized member of the kangaroo family, is a pouched mammal, or marsupial. The (b) echidna (Tachyglossus aculeatus) is an egg-laying mammal. (credit a: modification of work by Derrick Coetzee; credit b: modification of work by Allan Whittome.)
Sometimes ecologists discover unique patterns of species distribution by determining where species are not found. Hawaii, for example, has no native land species of reptiles or amphibians, and has only one native terrestrial mammal, the hoary bat. Most of New Guinea, as another example, lacks placental mammals.
Plants can be endemic or generalists: endemic plants are found only on specific regions of the Earth, while generalists are found on many regions. Isolated land masses—such as Australia, Hawaii, and Madagascar—often have large numbers of endemic plant species. Some of these plants are endangered due to human activity. The forest gardenia (Gardenia brighamii), for instance, is endemic to Hawaii; only an estimated 15–20 trees are thought to exist.
Energy Sources
Sunlight
Energy from the sun is captured by green plants, algae, cyanobacteria, and photosynthetic protists. These organisms convert solar energy into the chemical energy needed by all living things. Light availability can be an important force directly affecting the evolution of adaptations in photosynthesizers. For instance, plants in the understory of a temperate forest are shaded when the trees above them in the canopy completely leaf out in the late spring. Not surprisingly, understory plants have adaptations to successfully capture available light. One such adaptation is the rapid growth of spring ephemeral plants such as the spring beauty (Figure 14.3}. These spring flowers achieve much of their growth and finish their life cycle (reproduce) early in the season before the trees in the canopy develop leaves.
In aquatic ecosystems, the availability of light may be limited because sunlight is absorbed by water, plants, suspended particles, and resident microorganisms. Toward the bottom of a lake, pond, or ocean, there is a zone that light cannot reach. Photosynthesis cannot take place there and, as a result, a number of adaptations have evolved that enable living things to survive without light. For instance, aquatic plants have photosynthetic tissue near the surface of the water; for example, think of the broad, floating leaves of a water lily—water lilies cannot survive without light. In environments such as hydrothermal vents, some bacteria extract energy from inorganic chemicals because there is no light for photosynthesis.
The availability of nutrients in aquatic systems is also an important aspect of energy or photosynthesis. Many organisms sink to the bottom of the ocean when they die in the open water; when this occurs, the energy found in that living organism is sequestered for some time unless ocean upwelling occurs. Ocean upwelling is the rising of deep ocean waters that occurs when prevailing winds blow along surface waters near a coastline (Figure 14.4). As the wind pushes ocean waters offshore, water from the bottom of the ocean moves up to replace this water. As a result, the nutrients once contained in dead organisms become available for reuse by other living organisms.
In freshwater systems, the recycling of nutrients occurs in response to air temperature changes. The nutrients at the bottom of lakes are recycled twice each year: in the spring and fall turnover. The spring and fall turnover is a seasonal process that recycles nutrients and oxygen from the bottom of a freshwater ecosystem to the top of a body of water (Figure 14.5). These turnovers are caused by the formation of a thermocline: a layer of water with a temperature that is significantly different from that of the surrounding layers. In wintertime, the surface of lakes found in many northern regions is frozen. However, the water under the ice is slightly warmer, and the water at the bottom of the lake is warmer yet at 4 °C to 5 °C (39.2 °F to 41 °F). Water is densest at 4 °C; therefore, the deepest water is also the densest. The deepest water is oxygen poor because the decomposition of organic material at the bottom of the lake uses up available oxygen that cannot be replaced by means of oxygen diffusion into the water due to the surface ice layer.
How might turnover in tropical lakes differ from turnover in lakes that exist in temperate regions?
In springtime, air temperatures increase and surface ice melts. When the temperature of the surface water begins to reach 4 °C, the water becomes heavier and sinks to the bottom. The water at the bottom of the lake is then displaced by the heavier surface water and, thus, rises to the top. As that water rises to the top, the sediments and nutrients from the lake bottom are brought along with it. During the summer months, the lake water stratifies, or forms layers, with the warmest water at the lake surface.
As air temperatures drop in the fall, the temperature of the lake water cools to 4 °C; therefore, this causes fall turnover as the heavy cold water sinks and displaces the water at the bottom. The oxygen-rich water at the surface of the lake then moves to the bottom of the lake, while the nutrients at the bottom of the lake rise to the surface. During the winter, the oxygen at the bottom of the lake is used by decomposers and other organisms requiring oxygen, such as fish.
Temperature
Temperature affects the physiology of living things as well as the density and state of water. Temperature exerts an important influence on living things because few living things can survive at temperatures below 0 °C (32 °F) due to metabolic constraints. It is also rare for living things to survive at temperatures exceeding 45 °C (113 °F); this is a reflection of evolutionary response to typical temperatures. Enzymes are most efficient within a narrow and specific range of temperatures; enzyme degradation can occur at higher temperatures. Therefore, organisms either must maintain an internal temperature or they must inhabit an environment that will keep the body within a temperature range that supports metabolism. Some animals have adapted to enable their bodies to survive significant temperature fluctuations, such as seen in hibernation or reptilian torpor. Similarly, some bacteria are adapted to surviving in extremely hot temperatures such as geysers. Such bacteria are examples of extremophiles: organisms that thrive in extreme environments.
Temperature can limit the distribution of living things. Animals faced with temperature fluctuations may respond with adaptations, such as migration, in order to survive. Migration, the movement from one place to another, is an adaptation found in many animals, including many that inhabit seasonally cold climates. Migration solves problems related to temperature, locating food, and finding a mate. In migration, for instance, the Arctic Tern (Sterna paradisaea) makes a 40,000 km (24,000 mi) round trip flight each year between its feeding grounds in the southern hemisphere and its breeding grounds in the Arctic Ocean. Monarch butterflies (Danaus plexippus) live in the eastern United States in the warmer months and migrate to Mexico and the southern United States in the wintertime. Some species of mammals also make migratory forays. Reindeer (Rangifer tarandus) travel about 5,000 km (3,100 mi) each year to find food. Amphibians and reptiles are more limited in their distribution because they lack migratory ability. Not all animals that can migrate do so: migration carries risk and comes at a high energy cost.
Some animals hibernate or estivate to survive hostile temperatures. Hibernation enables animals to survive cold conditions, and estivation allows animals to survive the hostile conditions of a hot, dry climate. Animals that hibernate or estivate enter a state known as torpor: a condition in which their metabolic rate is significantly lowered. This enables the animal to wait until its environment better supports its survival. Some amphibians, such as the wood frog (Rana sylvatica), have an antifreeze-like chemical in their cells, which retains the cells’ integrity and prevents them from bursting.
Water
Water is required by all living things because it is critical for cellular processes. Since terrestrial organisms lose water to the environment by simple diffusion, they have evolved many adaptations to retain water.
-
-
-
- Plants have a number of interesting features on their leaves, such as leaf hairs and a waxy cuticle, that serve to decrease the rate of water loss via transpiration.
- Freshwater organisms are surrounded by water and are constantly in danger of having water rush into their cells because of osmosis. Many adaptations of organisms living in freshwater environments have evolved to ensure that solute concentrations in their bodies remain within appropriate levels. One such adaptation is the excretion of dilute urine.
- Marine organisms are surrounded by water with a higher solute concentration than the organism and, thus, are in danger of losing water to the environment because of osmosis. These organisms have morphological and physiological adaptations to retain water and release solutes into the environment. For example, Marine iguanas (Amblyrhynchus cristatus), sneeze out water vapor that is high in salt in order to maintain solute concentrations within an acceptable range while swimming in the ocean and eating marine plants.
-
-
Inorganic Nutrients and Soil
Inorganic nutrients, such as nitrogen and phosphorus, are important in the distribution and the abundance of living things. Plants obtain these inorganic nutrients from the soil when water moves into the plant through the roots. Therefore, soil structure (particle size of soil components), soil pH, and soil nutrient content play an important role in the distribution of plants. Animals obtain inorganic nutrients from the food they consume. Therefore, animal distributions are related to the distribution of what they eat. In some cases, animals will follow their food resource as it moves through the environment.
Other Aquatic Factors
Some abiotic factors, such as oxygen, are important in aquatic ecosystems as well as terrestrial environments. Terrestrial animals obtain oxygen from the air they breathe. Oxygen availability can be an issue for organisms living at very high elevations, however, where there are fewer molecules of oxygen in the air. In aquatic systems, the concentration of dissolved oxygen is related to water temperature and the speed at which the water moves. Cold water has more dissolved oxygen than warmer water. In addition, salinity, current, and tide can be important abiotic factors in aquatic ecosystems.
Other Terrestrial Factors
Wind can be an important abiotic factor because it influences the rate of evaporation and transpiration. The physical force of wind is also important because it can move soil, water, or other abiotic factors, as well as an ecosystem’s organisms.
Fire is another terrestrial factor that can be an important agent of disturbance in terrestrial ecosystems. Some organisms are adapted to fire and, thus, require the high heat associated with fire to complete a part of their life cycle. For example, the jack pine—a coniferous tree—requires heat from fire for its seed cones to open (Figure 14.6). Through the burning of pine needles, fire adds nitrogen to the soil and limits competition by destroying undergrowth.
Abiotic Factors Influencing Plant Growth
Temperature and moisture are important influences on plant production (primary productivity) and the amount of organic matter available as food (net primary productivity). Net primary productivity is an estimation of all of the organic matter available as food; it is calculated as the total amount of carbon fixed per year minus the amount that is oxidized during cellular respiration. In terrestrial environments, net primary productivity is estimated by measuring the aboveground biomass per unit area, which is the total mass of living plants, excluding roots. This means that a large percentage of plant biomass which exists underground is not included in this measurement. Net primary productivity is an important variable when considering differences in biomes. Very productive biomes have a high level of aboveground biomass.
Annual biomass production is directly related to the abiotic components of the environment. Environments with the greatest amount of biomass have conditions in which photosynthesis, plant growth, and the resulting net primary productivity are optimized. The climate of these areas is warm and wet. Photosynthesis can proceed at a high rate, enzymes can work most efficiently, and stomata can remain open without the risk of excessive transpiration; together, these factors lead to the maximal amount of carbon dioxide (CO2) moving into the plant, resulting in high biomass production. The aboveground biomass produces several important resources for other living things, including habitat and food. Conversely, dry and cold environments have lower photosynthetic rates and therefore less biomass. The animal communities living there will also be affected by the decrease in available food.
Movement in Biogeography
Living organisms must find suitable habitat in order to survive. One individual animal might be able, or need to, move short distances over a lifetime, perhaps only a few cm or m, while another individual may need to move 1000s of km. An individual plant won’t move on it’s own, but the species may move through seed dispersal. Think of the wings on a maple tree seed or the ‘stickiness’ of a cocklebur and how that helps the species find new suitable habitat.
Island Biogeography
Organisms and biological communities often vary in a regular fashion along geographic gradients of latitude, elevation, isolation, and habitat area. The latter pattern, often called the species-area relationship, has long been a fascination for biogeographers.
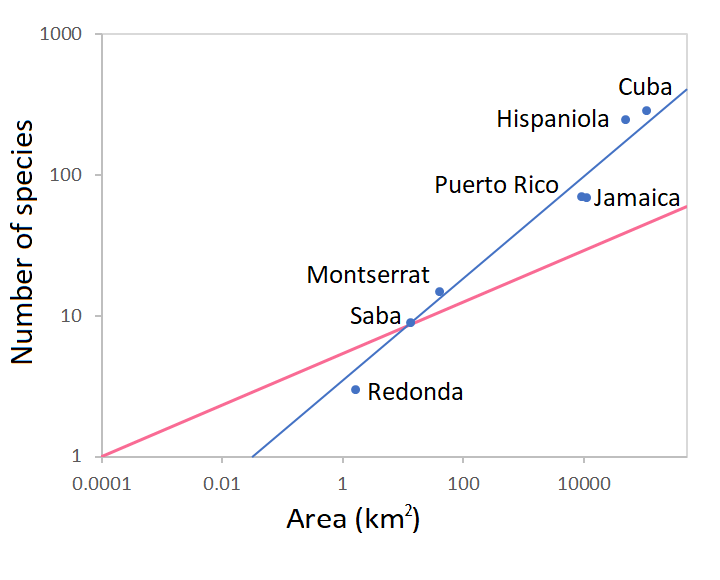
The figure below demonstrates this species-area relationship for amphibians and reptiles in the West Indies. A study from around 1957 was included in Robert H. MacArthur and Edward O. Wilson’s famous book “The Theory of Island Biogeography”, and showed that among seven islands studied, the largest, Cuba, had the most species, and the smallest, Redonda, had the fewest. This general pattern has been found in many different islands groups, and across various different taxa.
An original study (Darlington c. 1957), published in “The Theory of Island biogeography“, showed a strong positive correlation between island size and number of species (blue line). A more recent study by Gao and Perry (2016) included data for many additional small islands (smaller than Redonda) and found that while the pattern holds, the relationship is flatter than in the original study–indicated by the pink line. Figure drawn by Andy Wilson, based on Figure 4 in Gao and Perry (2016).
While studying these species-area relationships MacArthur and Wilson were drawn to another of the four geographic gradients–that of isolation. They observed that some large Islands had fewer species than would be expected for their size, while conversely, some small islands had more species than expected. These are exceptions to the species-area relationship. These patterns were explained by MacArthur and Wilson’s Equilibrium Theory of Island Biogeography, which takes into account the fact that islands are colonized by species from elsewhere, and natural extinctions will occur on those islands, over long periods of time. Imagine there are two islands located off the coast of the mainland. Although the two islands are about the same size, one island is located much farther away than island. If you are a bird that lives on the mainland, which island are you most likely to find? The answer is generally island. This means immigration (or colonization) is influenced by the distance of an island from the mainland (a source of colonists). Therefore, islands that are closer to the island are more likely to receive immigrants than islands that are further away. Two offshore islands–the Theory of Island Biogeography suggests that fewer species will colonize the more distant island a, in comparison to island b.
Once a species manages to reach and colonize an island, the rate of extinction is largely influenced by size of the island. This is because smaller islands tend to hold smaller populations (which are more likely to experience extinction due to stochastic effects like genetic drift). Larger habitat size reduces the probability of extinction of the colonized species due to chance events. Smaller islands are also likely to hold fewer populations in general because they have fewer resources and less diversity of resources. Larger islands have larger and more habitat areas, which typically leads to more differences in habitat or habitat heterogeneity. Higher heterogeneity means that there are more opportunities for a variety of species to find their suitable niches. Habitat heterogeneity also helps increase the number of species to successfully colonize after immigration. Now let’s consider the situation if we had two different islands sizes in our offshore archipelago, with, much bigger than. We would expect extinction rates to be much lower on islands than on the two smaller islands.
.png?revision=1&size=bestfit&width=589&height=471)
.png?revision=1&size=bestfit&width=641&height=512)
Stream Drift Paradox
The stream drift paradox looks at the distribution of individuals of a single species along a stream. Some insects in particular are poor swimmers in their aquatic juvenile stages and therefore tend to move downstream with the current rather than upstream against the current. The paradox is how did they arrive upstream to begin with? One theory is the adults of the species tend to hatch at a time of day with breezes going up the valley towards the headwaters. So the direction of movement depends on the life stage of the insect. There is a problem with this when you realize that not all species have a life stage out of the water (Humphries and Duxton, 2002).
Invasive Species
Invasion of a region by new species is normal. Species are constantly testing new areas as seeds are deposited and animals wander. What’s not normal is the number of species that are moving so far from their hearth regions. This change is because of human activity, deliberate or not. As humans more often, greater distances, at greater speed, there’s more opportunity for species to move with the humans.
The Washington Invasive Species Council monitors and coordinates projects related to invasive species in the state. For many years the executive coordinator of the council was a trained geographer.
Most species fail to survive in the new location. The conditions the species need for survival simply don’t exist there. However, once in a while, the right conditions do exist. The new location may have fewer predators or more food. As the new species thrives, it removes space and nutrients that would have been available to local indigenous species.
The zebra mussel is believed to have come to the US Great Lakes on a ship from the Black Sea region in 1988. In 10 years it spread to all of the Great Lakes and many tributaries as shown in the video below.
NUTRIENT CYCLING
Consider different nutrient cycles such as Nitrogen, Water, Phosphorus, Potassium and many others. Where are they stored, for how long? What are their paths of movement from storage to storage. Think in Systems: There are locations of long term storage and paths of movement between them.
Large scale movement of animals represent mass movement. The animals have mass. But represents chemical and nutrient movement. Imagine the skies and trees in the 1800s as carrier pigeons migrated and roosted and clear the land of food, but left behind manure. Salmon mature in the ocean and concentrate nutrients found in the ocean only to die in coastal rivers and release those nutrients far inland. Without that natural movement of nutrients, humans need to do the movement instead or risk diminished biological productivity.
CONCLUSION
Biogeography helps explain and interpret the existing distribution of species, how they arrived there, and how species continue to move.
CITATION OF THIS CHAPTER
This chapter comes from several sources.
- The portion up to ‘Movement in Biogeography’ comes for OpenStax with the following proviso:
This book may not be used in the training of large language models or otherwise be ingested into large language models or generative AI offerings without OpenStax’s permission.
Want to cite, share, or modify this book? This book uses the Creative Commons Attribution License and you must attribute OpenStax.
Attribution information
- If you are redistributing all or part of this book in a print format, then you must include on every physical page the following attribution:
Access for free at https://openstax.org/books/biology/pages/1-introduction
- If you are redistributing all or part of this book in a digital format, then you must include on every digital page view the following attribution:
Access for free at https://openstax.org/books/biology/pages/1-introduction
Citation information
- Use the information below to generate a citation. We recommend using a citation tool such as this one.
- Authors: Connie Rye, Robert Wise, Vladimir Jurukovski, Jean DeSaix, Jung Choi, Yael Avissar
- Publisher/website: OpenStax
- Book title: Biology
- Publication date: Oct 21, 2016
- Location: Houston, Texas
- Book URL: https://openstax.org/books/biology/pages/1-introduction
- Section URL: https://openstax.org/books/biology/pages/44-2-biogeography
© Feb 14, 2022 OpenStax. Textbook content produced by OpenStax is licensed under a Creative Commons Attribution License . The OpenStax name, OpenStax logo, OpenStax book covers, OpenStax CNX name, and OpenStax CNX logo are not subject to the Creative Commons license and may not be reproduced without the prior and express written consent of Rice University.
2) The ‘Island Biogegraphy’ subsection is from: Island Biogeography is originally from: 21.3: Island Biogeography is shared under a CC BY-NC-SA license and was authored, remixed, and/or curated by LibreTexts.
3) The subsections on Stream Drift, Invasive Species, and Nutrient cycling are largely created by Cameron McCormick.
Stuart Humphries and Graeme D. Ruxton Journal of Animal Ecology, Vol. 71, No. 1 (Jan., 2002), pp. 151-154 (4 pages)