9 Earth: Plate Tectonics
LEARNING OUTCOMES
- Compare and describe each of these Earth layers: lithosphere, oceanic crust, and continental crust.
- Describe how convection takes place in the mantle and compare the two parts of the core and describe why they are different from each other.
- Explain the concepts of the following hypothesis: continental drift hypothesis, seafloor spreading hypothesis, and the theory of plate tectonics.
- Describe the three types of tectonic plate and how the processes lead to changes in Earth’s surface features.
ESSENTIAL QUESTIONS
- What is the driving force of plate tectonics and how does this impact earthquakes and volcanoes around the world?
- How does the theory of plate tectonics help explain the different types of earthquakes and volcanoes around the planet?
UNDERSTANDING EARTH’S INTERIOR
Before you can learn about plate tectonics, you need to know something about the layers that are found inside Earth. These layers are divided by composition into core, mantle, and crust or by mechanical properties into lithosphere and asthenosphere. Scientists use information from earthquakes and computer modeling to learn about Earth’s interior. Humans have never drilled past Earth’s crust, and yet we know a lot about the composition of the earth’s interior. Rocks yield some clues, but they only reveal information about the outer crust. In rare instances, a mineral, such as a diamond, comes to the surface from deeper down in the crust or the mantle. To learn about Earth’s interior, scientists use energy, recorded by seismographs, to “see” the different layers of the Earth, just like doctors can use an MRI, CT scan, or x-ray to see inside our bodies.
SEISMIC WAVES
One ingenious way scientists learn about Earth’s interior is by looking at how energy travels from the point of an earthquake, called seismic waves. Seismic waves travel outward in all directions from where the ground breaks at an earthquake. Seismograph stations measure the energy released by these earthquakes, but there are two that scientists are most interested with in regards to understanding the interior of the earth.
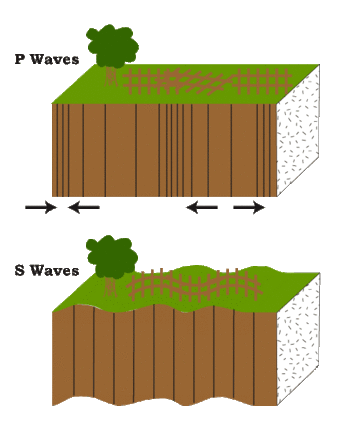
Primary waves (also called P-waves) are fastest, traveling at about 6 to 7 kilometers (about 4 miles) per second, so they arrive first at the seismometer. P-waves move in a compression/expansion type motion, squeezing and unsqueezing earth materials as they travel. P-waves bend slightly when they travel from one layer into another. Seismic waves move faster through denser or more rigid material. As P-waves encounter the liquid outer core, which is less rigid than the mantle, they slow down. This makes the P-waves arrive later and further away than would be expected. The result is a P-wave shadow zone. No P-waves are picked up at seismographs 104° to 140° from the earthquakes focus.
Secondary waves (also called S-waves) are about half as fast as P-waves, traveling at about 3.5 km (2 miles) per second, and arrive second at seismographs. S-waves move in an up and down motion perpendicular to the direction of wave travel. This produces a change in shape for the earth materials they move through. Only solids resist a change in shape, so S-waves are only able to propagate through solids. S-waves cannot travel through liquid. By tracking seismic waves, scientists have learned what makes up the planet’s interior. P-waves slow down at the mantle core boundary, so we know the outer core is less rigid than the mantle. S-waves disappear at the mantle core boundary, so the outer core is liquid.
Other clues to Earth’s interior includes the fact that we know that Earth’s overall density is higher than the density of crustal rocks, so the core must be made of something dense, like metal. Also, since Earth has a magnetic field, there must be metal within the planet. Iron and nickel are both magnetic. Finally, meteorites are the remains of the material that formed the early solar system and are thought to be similar to material in Earth’s interior.
COMPOSITION AND STRUCTURE OF EARTH
Core, mantle, and crust are divisions based on composition. The crust makes up less than 1 percent of Earth by mass, consisting of oceanic crust and continental crust is often more felsic rock. The mantle is hot and represents about 68 percent of Earth’s mass. Finally, the core is mostly iron metal. The core makes up about 31% of the Earth. The lithosphere and asthenosphere are divisions based on mechanical properties. The lithosphere is composed of both the crust and the portion of the upper mantle that behaves as a brittle, rigid solid. The asthenosphere is partially molten upper mantle material that behaves plastically and can flow.
CRUST AND LITHOSPHERE
Earth’s outer surface is its crust; a cold, thin, brittle outer shell made of rock. The crust is very thin, relative to the radius of the planet. There are two very different types of crust, each with its own distinctive physical and chemical properties.
Oceanic crust is composed of magma that erupts on the seafloor to create basalt lava flows or cools deeper down to create the intrusive igneous rock gabbro. Sediments, primarily muds and the shells of tiny sea creatures, coat the seafloor. Sediment is thickest near the shore where it comes off the continents in rivers and on wind currents. Ocean plates are rich in iron.
Continental crust is made up of many different types of igneous, metamorphic, and sedimentary rocks. The average composition is granite, which is much less dense than the mafic igneous rocks of the oceanic crust. Because it is thick and has relatively low density, continental crust rises higher on the mantle than oceanic crust, which sinks into the mantle to form basins. When filled with water, these basins form the planet’s oceans. Continental plates are rich in silicates and have relatively less iron than ocean plates.
The lithosphere is the outermost mechanical layer, which behaves as a brittle, rigid solid. The lithosphere is about 100 kilometers thick. The definition of the lithosphere is based on how earth materials behave, so it includes the crust and the uppermost mantle, which are both brittle. Since it is rigid and brittle, when stresses act on the lithosphere, it breaks. This is what we experience as an earthquake.
MANTLE
The two most important things about the mantle are: (1) it is made of solid rock, and (2) it is hot. Scientists know that the mantle is made of rock based on evidence from seismic waves, heat flow, and meteorites. The properties fit the ultramafic rock peridotite, which is made of the iron- and magnesium-rich silicate minerals. Peridotite is rarely found at Earth’s surface.
Scientists know that the mantle is extremely hot because of the heat flowing outward from it and because of its physical properties. Heat flows in two different ways within the Earth: conduction and convection. Conduction is defined as the heat transfer that occurs through rapid collisions of atoms, which can only happen if the material is solid. Heat flows from warmer to cooler places until all are the same temperature. The mantle is hot mostly because of heat conducted from the core. Convection is the process of a material that can move and flow may develop convection currents.
Convection in the mantle is the same as convection in a pot of water on a stove. Convection currents within Earth’s mantle form as material near the core heats up. As the core heats the bottom layer of mantle material, particles move more rapidly, decreasing its density and causing it to rise. The rising material begins the convection current. When the warm material reaches the surface, it spreads horizontally. The material cools because it is no longer near the core. It eventually becomes cool and dense enough to sink back down into the mantle. At the bottom of the mantle, the material travels horizontally and is heated by the core. It reaches the location where warm mantle material rises, and the mantle convection cell is complete.
Convection in the mantle is the same as convection in a pot of water on a stove. Convection currents within Earth’s mantle form as material near the core heats up. As the core heats the bottom layer of mantle material, particles move more rapidly, decreasing its density and causing it to rise. The rising material begins the convection current. When the warm material reaches the surface, it spreads horizontally. The material cools because it is no longer near the core. It eventually becomes cool and dense enough to sink back down into the mantle. At the bottom of the mantle, the material travels horizontally and is heated by the core. It reaches the location where warm mantle material rises, and the mantle convection cell is complete.
CORE
At the planet’s center lies a dense metallic core. Scientists know that the core is metal for a few reasons. The density of Earth’s surface layers is much less than the overall density of the planet, as calculated from the planet’s rotation. If the surface layers are less dense than average, then the interior must be denser than average. Calculations indicate that the core is about 85 percent iron metal with nickel metal making up much of the remaining 15 percent. Also, metallic meteorites are thought to be representative of the core.
If Earth’s core were not metal, the planet would not have a magnetic field. Metals such as iron are magnetic, but rock, which makes up the mantle and crust, is not. Scientists know that the outer core is liquid and the inner core is solid because S-waves stop at the inner core. The strong magnetic field is caused by convection in the liquid outer core. Convection currents in the outer core are due to heat from the even hotter inner core. The heat that keeps the outer core from solidifying is produced by the breakdown of radioactive elements in the inner core.
CONTINENTAL DRIFT
The continental drift hypothesis was developed in the early part of the 20th century, mostly by Alfred Wegener. Wegener said that continents move around on Earth’s surface and that they were once joined together as a single supercontinent. While Wegener was alive, scientists did not believe that the continents could move.
Find a map of the continents and cut each one out. Better yet, use a map where the edges of the continents show the continental shelf. That’s the true size and shape of a continent and many can be pieced together like a puzzle. The easiest link is between the eastern Americas and western Africa and Europe, but the rest can fit together too.
Alfred Wegener proposed that the continents were once united into a single supercontinent named Pangaea, meaning all earth in ancient Greek. He suggested that Pangaea broke up long ago and that the continents then moved to their current positions. He called his hypothesis continental drift.
EVIDENCE FOR CONTINENTAL DRIFT
Besides the way the continents fit together, Wegener and his supporters collected a great deal of evidence for the continental drift hypothesis. For one, identical rocks of the same type and age are found on both sides of the Atlantic Ocean. Wegener said the rocks had formed side-by-side and that the land had since moved apart.
Mountain ranges with the same rock types, structures, and ages are now on opposite sides of the Atlantic Ocean. The Appalachians of the eastern United States and Canada, for example, are just like mountain ranges in eastern Greenland, Ireland, Great Britain, and Norway. Wegener concluded that they formed as a single mountain range that was separated as the continents drifted.
Ancient fossils of the same species of extinct plants and animals are found in rocks of the same age but are on continents that are now widely separated. Wegener proposed that the organisms had lived side by side, but that the lands had moved apart after they were dead and fossilized. He suggested that the organisms would not have been able to travel across the oceans. For example, the fossils of the seed fern Glossopteris were too heavy to be carried so far by wind. The reptile Mesosaurus could only swim in fresh water. was a swimming reptile but could only swim in fresh water. Cynognathus and Lystrosaurus were land reptiles and were unable to swim.
Grooves and rock deposits left by ancient glaciers are found today on different continents very close to the equator. This would indicate that the glaciers either formed in the middle of the ocean and/or covered most of the Earth. Today glaciers only form on land and nearer the poles. Wegener thought that the glaciers were centered over the southern land mass close to the South Pole and the continents moved to their present positions later on.
Coral reefs and coal-forming swamps are found in tropical and subtropical environments, but ancient coal seams and coral reefs are found in locations where it is much too cold today. Wegener suggested that these creatures were alive in warm climate zones and that the fossils and coal later had drifted to new locations on the continents.
Although Wegener’s evidence was sound, most geologists at the time rejected his hypothesis of continental drift. Scientists argued that there was no way to explain how solid continents could plow through solid oceanic crust. There was no mechanism explaining how the continents moved. Wegener’s idea was nearly forgotten until technological advances presented even more evidence that the continents moved and gave scientists the tools to develop a mechanism for Wegener’s drifting continents.
THE SEAFLOOR
Wegener first proposed his concept of Continental Drift in 1915. There weren’t effective tools available for investigating the ocean floor. But that changed during and after WWII. World War II gave scientists the tools to find the mechanism for continental drift that had eluded Wegener. Maps and other data gathered during the war allowed scientists to develop the seafloor spreading hypothesis. This hypothesis traces oceanic crust from its origin at a mid-ocean ridge to its destruction at a deep sea trench and is the mechanism for continental drift.
Mapping the Ocean Floors: Physical geography
During World War II, battleships and submarines carried echo sounders to locate enemy submarines. Echo sounders produce sound waves that travel outward in all directions, bounce off the nearest object, and then return to the ship. By knowing the speed of sound in seawater, scientists calculate the distance to the object based on the time it takes for the wave to make a round-trip. During the war, most of the sound waves ricocheted off the ocean bottom.
After the war, using sonar information, Bruce Heezen and Marie Tharp created the first detailed map of the ocean floor to reveal the Mid-Atlantic Ridge, a basaltic mountain range that spanned the length of the Atlantic Ocean, with dimensions unlike the mountains found on the continents. The world now had a new understanding of the shape and landforms of the seafloor.
The next step is to explain those landforms. Initially scientists thought the ridge was part of a mechanism that explained the expanding Earth or ocean-basin growth hypotheses. In 1959, Harry Hess proposed the hypothesis of seafloor spreading – that the mid-ocean ridges represented tectonic plate factories, where new oceanic plate was issuing from these long volcanic ridges. Scientists later included transform faults perpendicular to the ridges to better account for varying rates of movement between the newly formed plates. When earthquake epicenters were discovered along the ridges, the idea that earthquakes were linked to plate movement took hold.
Seafloor sediment, measured by dredging and drilling, provided another clue. Scientists once believed sediment accumulated on the ocean floors over a very long time in a static environment. When some studies showed less sediment than expected, these results were initially used to argue against continental movement. With more time, researchers discovered these thinner sediment layers were located close to mid-ocean ridges, indicating the ridges were younger than the surrounding ocean floor. This finding supported the idea that the sea floor was not fixed in one place.
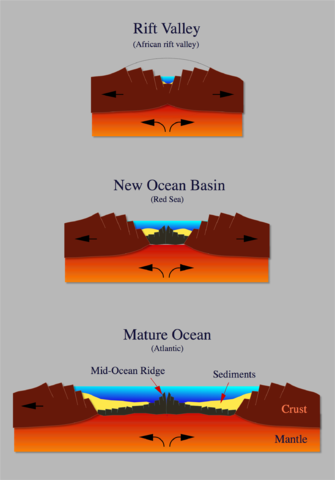
Explaining the landforms: Chemistry and Magnetism
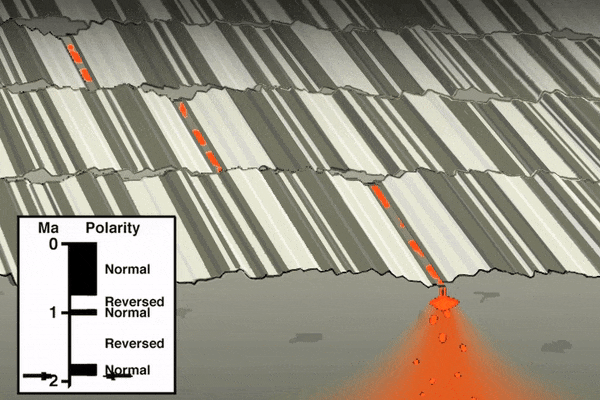
During WWII, magnetometers devices capable of measuring the magnetic field intensity, were also attached to ships to search for submarines. They found an astonishing feature. The magnetic polarity of seafloor basalts reverses, and creates a pattern. Stripes of normal polarity and reversed polarity alternate across the ocean bottom. These stripes also forms a mirror image of itself on either side of the mid-ocean ridges. But the stripes end abruptly at the edges of continents, sometimes at a deep sea trench.
Although the magnetite crystals were pointing to the magnetic north pole, the location of the pole seemed to wander. Scientists were amazed to find that the north magnetic pole changed location through time. There are three possible explanations for this: 1) The continents remained fixed and the north magnetic pole moved. 2) The north magnetic pole stood still and the continents moved, or 3) both the continents and the north pole moved.
Geologists noted that for rocks of the same age but on different continents, the little magnets pointed to different magnetic north poles. For example, 400-million-year-old magnetite in Europe pointed to a different north magnetic pole than the same-aged magnetite in North America. Around 250 million years ago, the north poles were also different for the two continents.
The scientists looked again at the three possible explanations. Only one can be correct. If the continents had remained fixed while the north magnetic pole moved, there must have been two separate north poles. Since there is only one north pole today, the only reasonable explanation is that the north magnetic pole has remained fixed but that the continents have moved.
To test this, geologists fitted the continents together as Wegener had done and behold, it worked. There has only been one magnetic north pole and the continents have drifted. They named the phenomenon of the magnetic pole that seemed to move but actually did not apparent polar wander. This evidence for continental drift gave geologists renewed interest in understanding how continents could move about on the planet’s surface.

The oldest seafloor is near the edges of continents or deep sea trenches and is less than 180 million years old. Since the oldest ocean crust is so much younger than the oldest continental crust, scientists realized that seafloor was being destroyed in a relatively short time.
SEAFLOOR SPREADING HYPOTHESIS, How Plates Move
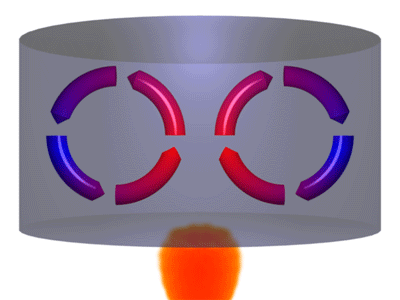
Scientists brought these observations together in the early 1960s to create the seafloor spreading hypothesis. In this hypothesis, hot buoyant mantle rises up a mid-ocean ridge, causing the ridge to rise upward. The hot magma at the ridge erupts as lava that forms new seafloor. When the lava cools, the magnetite crystals take on the current magnetic polarity and as more lava erupts, it pushes the seafloor horizontally away from ridge axis.
The magnetic stripes continue across the seafloor. As oceanic crust forms and spreads, moving away from the ridge crest, it pushes the continent away from the ridge axis. If the oceanic crust reaches a deep sea trench, it sinks into the trench and is lost into the mantle. Scientists now know that the oldest crust is coldest and lies deepest in the ocean because it is less buoyant than the hot new crust.
Seafloor spreading is the mechanism for Wegener’s drifting continents. Convection currents within the mantle take the continents on a conveyor-belt ride of oceanic crust that over millions of years takes them around the planet’s surface.
TECTONIC THEORY
Plate tectonics is the unifying theory of geology. Plate tectonics theory explains why:
- Earth’s geography has changed through time and continues to change today.
- some places are prone to earthquakes while others are not.
- certain regions may have deadly, mild, or no volcanic eruptions.
- mountain ranges are located where they are.
Plate tectonic motions affect Earth’s rock cycle, climate, and the evolution of life.
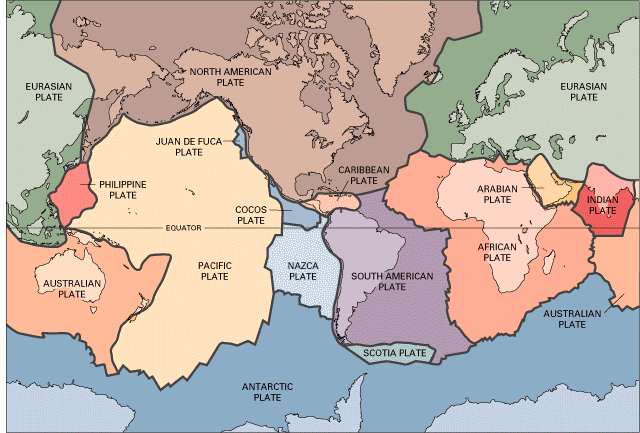
PLATE BOUNDARIES
We’ve now looked at the interior of the Earth, the major landforms of the surface, and how plates move. Now let’s see what happens at the surface because of the movement and interaction of different plates.
Plate boundaries are the edges where two plates meet. Most geologic activities, including volcanoes, earthquakes, and mountain building, take place at plate boundaries. How can two plates move relative to each other?
- Divergent plate boundaries: the two plates move away from each other.
- Convergent plate boundaries: the two plates move towards each other.
- Transform plate boundaries: the two plates slip past each other.
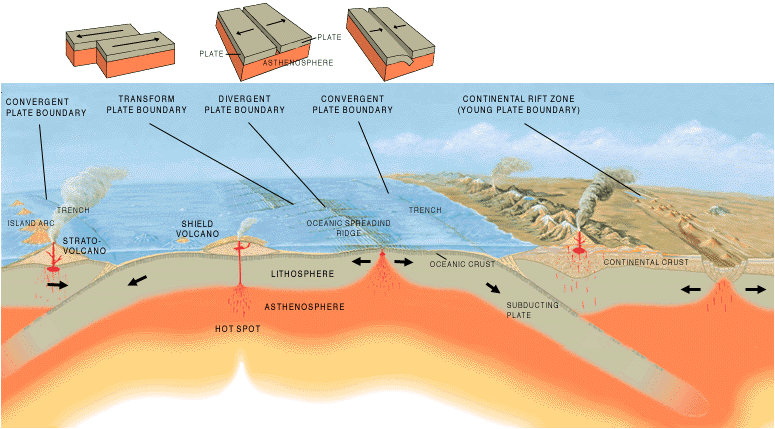
Schematic of plate boundary types.
The type of plate boundary and the type of crust found on each side of the boundary determines what sort of geologic activity will be found there.
Plate Boundary Landforms by the Numbers
1 Earth
2 Types of Plates: Oceanic and Continental
3 Types of Relative Movement: Divergent, Convergent, Transform
2×3= 6 Unique Landforms: Non-Volcanic Mountains, Volcanoes, Trenches, Ocean Ridges, Continental Rifts, Narrow Valleys
DIVERGENT PLATE BOUNDARIES

Plates move apart at mid-ocean ridges where new seafloor forms. Between the two plates is a rift valley. Lava flows at the surface cool rapidly to become basalt, but deeper in the crust, magma cools more slowly to form gabbro. So the entire ridge system is made up of igneous rock that is either extrusive or intrusive. Earthquakes are common at mid-ocean ridges since the movement of magma and oceanic crust results in crustal shaking. The vast majority of mid-ocean ridges are located deep below the sea. Here is an animation from the United States Geologic Survey (USGS) of divergent plate boundary at mid-ocean ridge and another animation by IRIS.As divergence occurs, shallow earthquakes can occur along with volcanoes along the rift areas. When the process begins, a valley will develop such as the Great Rift Valley in Africa. Over time that valley can fill up with water creating linear lakes. If divergence continues, a sea can form like the Red Sea and finally an ocean like the Atlantic Ocean. Check out the eastern half of Africa and notice the lakes that look linear. Eastern Africa is tearing apart from these linear lakes, to the Great Rift Valley, and up to the Red Sea. The ultimate divergent boundary is the Atlantic Ocean, which began when Pangea broke apart.
CONVERGENT PLATE BOUNDARIES

When two plates converge, the result depends on the type of lithosphere the plates are made of. No matter what, smashing two enormous slabs of lithosphere together results in the creation of magma and earthquakes.
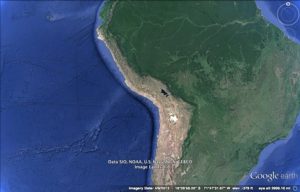
Ocean-to-continent convergence occurs when oceanic crust converges with continental crust, forcing the denser oceanic plate to plunge beneath the continental plate. This process called subduction, occurs along oceanic trenches called subduction zones where lots of intense earthquakes and volcanic eruptions can occur. The denser, subducting plate begins to heat up under extreme pressure near the mantle and melts to create causes melting in the volcanoes. These coastal volcanic mountains are found in a line above the subducting plate. The volcanoes are known as a continental arc. The movement of crust and magma causes earthquakes. Click here for a map of earthquake epicenters at subduction zones.
The volcanoes of northeastern California—Lassen Peak, Mount Shasta, and Medicine Lake volcano—along with the rest of the Cascade Mountains of the Pacific Northwest are the result of subduction of the Juan de Fuca plate beneath the North American plate. The Juan de Fuca plate is created by seafloor spreading just offshore at the Juan de Fuca ridge.
If the magma at a continental arc is felsic, it may be too viscous (thick) to rise through the crust. The magma will cool slowly to form granite or granodiorite. These large bodies of intrusive igneous rocks are called batholiths, which may someday be uplifted to form a mountain range.
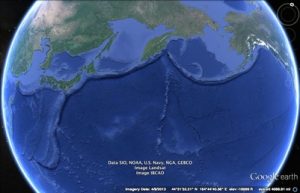
An oceanic-to-oceanic plate boundary occurs when two oceanic plates converge, causing the older, denser plate will subduct into the mantle. An ocean trench marks the location where the plate is pushed down into the mantle. The line of volcanoes that grows on the upper oceanic plate is an island arc.
The Ring of Fire is a ring around the Pacific Ocean of subduction zones, which most are oceanic-to-oceanic convergence. Here is an animation of an ocean continent plate boundary. Along these subduction zones, volcanic islands (also called volcanic arcs) form. Examples of these regions include Japan, Indonesia, and the Aleutian Islands.
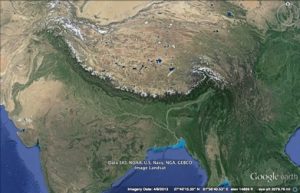
When two continental plates converge, instead of subduction, the two similar tectonic plates will buckle up to create large mountain ranges like a massive car pile-up. This is called continental-to-continental convergence, and geologically creates intense folding and faulting rather than volcanic activity.
Examples of mountain ranges created by this process are the Himalayan mountains as India is colliding with Asia, the Alps in Europe, and the Appalachian mountains in the United States as the North American plate collided with the African plate when Pangea was forming. The Kashmir India earthquake of 2005 that killed over 80,000 people occurred because of this process. And most recently, the 2008 earthquake in China which killed nearly 85,000 people before the Summer Olympics was because of this tectonic force. The Appalachian Mountains are the remnants of a large mountain range that was created when North America rammed into Eurasia about 250 million years ago.
TRANSFORM PLATE BOUNDARIES
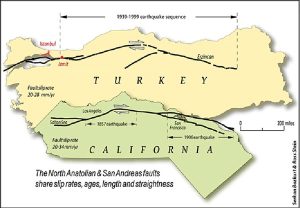
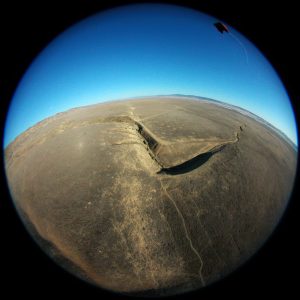
INTRAPLATE BOUNDARIES
A small amount of geologic activity, known as intraplate activity, does not take place at plate boundaries but within a plate instead. Mantle plumes are pipes of hot rock that rise through the mantle. The release of pressure causes melting near the surface to form a hotspot. Eruptions at the hotspot create a volcano. Hotspot volcanoes are found in a line. Can you figure out why? Hint: The youngest volcano sits above the hotspot and volcanoes become older with distance from the hotspot. Geologists use some hotspot chains to tell the direction and the speed a plate is moving. Hotspot magmas rarely penetrate through thick continental crust. One exception is the Yellowstone hotspot.
A CHANGING SURFACE
Geologists know that Wegener was right because the movements of continents explain so much about the geology we see. Most of the geologic activity that we see on the planet today is because of the interactions of the moving plates.
In the map of North America, where are the mountain ranges located? Using what you have learned about plate tectonics, try to answer the following questions:
- What is the geologic origin of the Cascades Range? The Cascades are a chain of volcanoes in the Pacific Northwest. They are not labelled on the diagram but they lie between the Sierra Nevada and the Coastal Range.
- What is the geologic origin of the Sierra Nevada? (Hint: These mountains are made of granitic intrusions.)
- What is the geologic origin of the Appalachian Mountains along the Eastern US?
Remember that Wegener used the similarity of the mountains on the west and east sides of the Atlantic as evidence for his continental drift hypothesis. The Appalachian mountains formed at a convergent plate boundary as Pangaea came together.
Before Pangaea came together, the continents were separated by an ocean where the Atlantic is now. The proto-Atlantic ocean shrank as the Pacific ocean grew. Currently, the Pacific is shrinking as the Atlantic is growing. This supercontinent cycle is responsible for most of the geologic features that we see and many more that are long gone.