10 Tectonic Forces: Quakes and Volcanoes
LEARNING OUTCOMES
- Describe the causes of tectonic stress and how they influence earthquakes and volcanoes.
- Understand where earthquakes and volcanoes are located around the world and why.
- Explain geologic structures that lead to folding and faulting of the physical landscape.
- Determine how the composition of magma determines the explosiveness of the volcanic eruption.
- Describe the various types of fault lines and volcanoes and the landforms they form.
ESSENTIAL QUESTIONS
- Could humans live in places along the Wasatch Front without the Wasatch fault and the earthquakes it causes?
- How does plate tectonics explain where and why earthquakes and volcanoes occur where they do?
- What role do earthquakes and volcanoes have on civilization? What benefits do they bring us?
- How could understanding Earth’s tectonic forces help save lives?
- What beneficial roles do tectonic forces have ecologically and for humanity?
TECTONIC STRESS
Three stresses work to deform the lithosphere and create a landscape of diverse landforms. Tectonic forces have the ability to lift rock and increase the elevation of the land surface, or ocean floor. In a later chapter we’ll discuss weathering and erosion which counteract tectonic forces. For now we’ll begin by considering what can happen to rocks when they are exposed to stress. Stress is the force applied to an object. In geosciences, stress is the force per unit area that is placed on a rock.
- Compression squeezes rocks together, causing rocks to fold or fracture. Compression is the most common stress at convergent plate boundaries.
- Rocks that are pulled apart are under tension. Rocks under tension lengthen or break apart. Tension is the major type of stress at divergent plate boundaries.
- When forces are parallel but moving in opposite directions, the stress is called shear. Shear stress is the most common stress at transform plate boundaries.
A rock’s response to stress depends on the rock type, the surrounding temperature, and pressure conditions the rock is under, the length of time the rock is under stress, and the type of stress. The rocks then have three possible responses to increasing stress: elastic deformation, plastic deformation, or fracturing. Elastic deformation occurs when the rock returns to its original shape when the stress is removed. When rocks under stress do not return to its original shape when the stress is removed, it is called plastic deformation. Finally, when a rock under stress breaks, it’s called a fracture.
Under what conditions do you think a rock is more likely to fracture? Is it more likely to break deep within Earth’s crust or at the surface? What if the stress applied is sharp rather than gradual? At the Earth’s surface, rocks usually break quite quickly, but deeper in the crust, where temperatures and pressures are higher, rocks are more likely to deform plastically. Sudden stress, such as a hit with a hammer, is more likely to make a rock break. Stress applied over time often leads to plastic deformation.
Geologic Structures
Sedimentary rocks are important for deciphering the geologic history of a region because they follow certain rules. First, sedimentary rocks are formed with the oldest layers on the bottom and the youngest on top. Second, sediments are deposited horizontally, so sedimentary rock layers are originally horizontal, as are some volcanic rocks, such as ash falls. Finally, sedimentary rock layers that are not horizontal are deformed in some manner. Often times looking like they are tiling into the earth.
You can trace the deformation a rock has experienced by seeing how it differs from its original horizontal, oldest-on-bottom position. This deformation produces geologic structures such as folds, joints, and faults that are caused by stresses.
FOLDS
Rocks deforming plastically under compressive stresses crumple into folds. They do not return to their original shape. If the rocks experience more stress, they may undergo more folding or even fracture. There are three major types of rock folding: monoclines, synclines, and anticlines. A monocline is a simple bend in the rock layers so that they are no longer horizontal. Anticlines are folded rocks that arch upward and dip away from the center of the fold. The oldest rocks are at the center of an anticline and the youngest are draped over them. When rocks arch upward to form a circular structure, that structure is called a dome. A syncline is a fold that bends downward, causing the youngest rocks are to be at the center and the oldest are on the outside. When rocks bend downward in a circular structure, that structure is called a basin. If the rocks are exposed at the surface, where are the oldest rocks located?

FAULTS
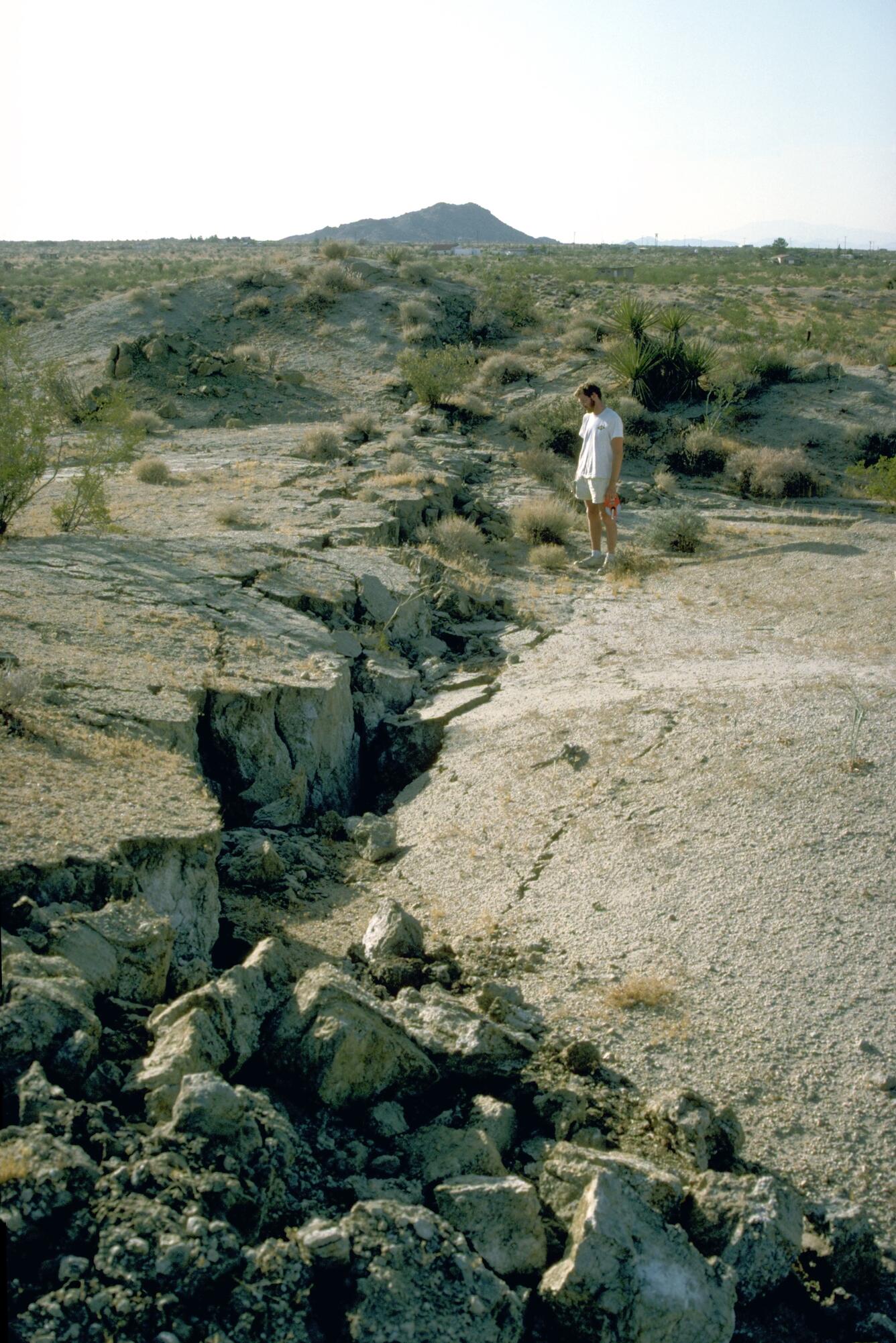
A rock under enough stress will eventually fracture. If there is no movement on either side of a fracture, the fracture is called a joint. But if the blocks of rock on one or both sides of a fracture move, the fracture is called a fault. Sudden motions along faults cause rocks to break and move suddenly, releasing the stored up stress energy to create an earthquake.
A slip is the distance rocks move along a fault and can be up or down the fault plane. Slip is relative, because there is usually no way to know whether both sides moved or only one. Faults lie at an angle to the horizontal surface of the Earth. That angle is called the fault’s dip. The dip defines which of two basic types a fault is. If the fault’s dip is inclined relative to the horizontal, the fault is a dip-slip fault. There are two types of dip-slip faults. In normal faults, the hanging wall drops down relative to the footwall. Normal faults can be huge and are often times responsible for uplifting mountain ranges in regions experiencing tensional stress.
With reverse faults, the footwall drops down relative to the hanging wall. A type of reverse fault is a thrust fault, in which the fault plane angle is nearly horizontal. Rocks can slip many miles along thrust faults.
A strike-slip fault is a dip-slip fault in which the dip of the fault plane is vertical and result from shear stresses. California’s San Andreas Fault is the world’s most famous strike-slip fault. It is a right-lateral strike slip fault.
STRESS AND MOUNTAIN BUILDING
It is the shear power and strength of two or more converging continental plates smash upwards that create mountain ranges. Stresses from this uplift cause folds, reverse faults, and thrust faults, which allow the crust to rise upwards. Subduction of oceanic lithosphere at convergent plate boundaries also builds mountain ranges.
When tensional stresses pull crust apart, it breaks into blocks that slide up and drop down along normal faults. The result is alternating mountains and valleys, known as a basin-and-range.
EARTHQUAKES
CAUSES OF QUAKES
An earthquake is sudden ground movement caused by the sudden release of energy stored in rocks, called the elastic rebound theory. Earthquakes happen when so much stress builds up in the rocks that the rocks rupture. The energy is transmitted by seismic waves. Each year there are more than 150,000 earthquakes strong enough to be felt by people and 900,000 recorded by seismometers!
In an earthquake, the initial point where the rocks rupture in the crust is called the focus (sometimes called the hypocenter). The epicenter is the point on the land surface that is directly above the focus. In about 75% of earthquakes, the focus is in the top 10 to 15 kilometers (6 to 9 miles) of the crust. Shallow earthquakes cause the most damage because the focus is near where people live. However, it is the epicenter of an earthquake that is reported by scientists and the media.
SEISMIC WAVES
Physics shows us that energy is always transmitted in waves. Every wave has a high point called a crest and a low point called a trough. The height of a wave from the center line to its crest is its amplitude. The distance between waves from crest to crest (or trough to trough) is its wavelength.
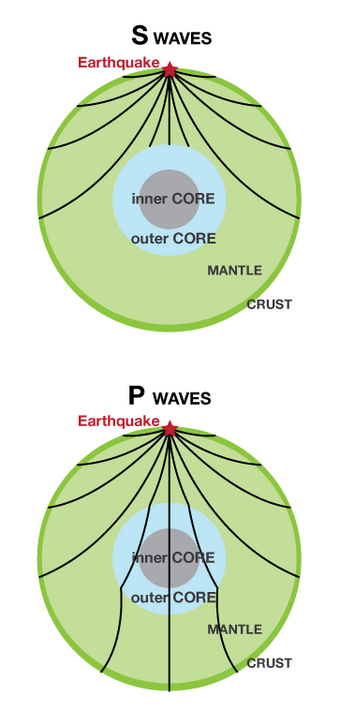
The energy from earthquakes travels in seismic waves. The study of seismic waves is known as seismology. Seismologists use seismic waves to learn about earthquakes and also to learn about the Earth’s interior. The two types of seismic waves described in “Plate Tectonics,” P-waves and S-waves, are known as body waves because they move through the solid body of the Earth. P-waves travel through solids, liquids, and gases. S-waves only move through solids. Surface waves travel along the ground, outward from an earthquake’s epicenter. Surface waves are the slowest of all seismic waves, traveling at 2.5 km (1.5 miles) per second. In an earthquake, body waves produce sharp jolts, while rolling motions of surface waves do most of the damage in an earthquake.
LOCATING A QUAKE
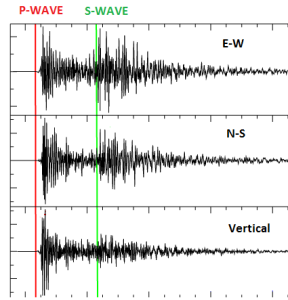
In order to locate an earthquake epicenter, scientists must first determine the epicenter distance from three different seismographs. P waves travel faster than S waves, so a seismogram will record P waves first, then S waves later. The longer the time between the arrival of the P-wave and S-wave, the farther away is the epicenter. So the difference in the P and S wave arrival times determines the distance between the epicenter and a seismometer. After analyzing thousands, perhaps millions of quakes, we now have a reliable estimate of the average speed of P and S ways.
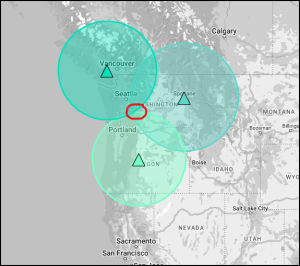
The scientist then draws a circle with a radius equal to the distance from the epicenter for that seismograph. The epicenter is somewhere along that circle. This is done for three locations. Using data from two seismographs, the two circles will intercept at two points. A third circle will intercept the other two circles at a single point. This point is the earthquake epicenter. Although useful for decades, this technique has been replaced by digital calculations. IRIS has an app here for practicing triangulation.
|
![]() |
MEASURING MAGNITUDE
People have always tried to quantify the size of and damage done by earthquakes. With the invention of the seismograph station, the Richter magnitude scale was created. Developed in 1935 by Charles Richter, this scale uses a seismometer to measure the magnitude of the largest jolt of energy released by an earthquake. Today, the moment magnitude scale has replaced the Richter scale. The moment magnitude scale measures the total energy released by an earthquake. Moment magnitude is calculated from the area of the fault that is ruptured and the distance the ground moved along the fault. The Richter scale and the moment magnitude scale are logarithmic. The amplitude of the largest wave increases ten times from one integer to the next. An increase in one integer means that thirty times more energy was released. These two scales often give very similar measurements. How does the amplitude of the largest seismic wave of a magnitude 5 earthquake compare with the largest wave of a magnitude 4 earthquake? How does it compare with a magnitude 3 quake? The amplitude of the largest seismic wave of a magnitude 5 quake is 10 times that of a magnitude 4 quake and 100 times that of a magnitude 3 quake.
A seismograph produces a graph-like representation of the seismic waves it receives and records them onto a seismogram. Seismograms contain information that can be used to determine how strong an earthquake was, how long it lasted, and how far away it was. Modern seismometers record ground motions using electronic motion detectors. The data are then kept digitally on a computer.
The third and oldest of the scale for quantifying quakes is the Mercalli Intensity scale. Earthquakes are described in terms of what nearby residents felt and the damage that was done to nearby structures. This scale is more qualitative in information because it’s based on visual damage and not the actual energy released by the earthquake. Today these maps are still important and various seismological stations will create shake maps of the surface damage.

Each scale has their benefits. As mentioned above, the Mercalli Intensity scale is based on how much damage someone would see. This is relative though because some places have strong building codes, and the rock material underneath will impact ground shaking without changing the energy released at the focus. With the Richter scale, a single sharp jolt measures higher than a very long intense earthquake that releases more energy. The moment magnitude scale more accurately reflects the energy released and the damage caused. Today, most seismologists now use the moment magnitude scale.
If a seismogram records P-waves and surface waves but not S-waves, the seismograph was on the other side of the Earth from the earthquake because those waves cannot travel through the liquid core of the earth. The amplitude of the waves can be used to determine the magnitude of the earthquake, which will be discussed in a later section.
In a single year, on average, more than 900,000 earthquakes are recorded and 150,000 of them are strong enough to be felt. Each year about 18 earthquakes are major with a Richter magnitude of 7.0 to 7.9, and on average one earthquake has a magnitude of 8 to 8.9.Magnitude 9 earthquakes are rare. The United States Geological Survey lists five since 1900. All but the Great Indian Ocean Earthquake of 2004 occurred somewhere around the Pacific Ocean basin.
LIVING WITH QUAKES
Scientists are a long way from being able to predict earthquakes. A good prediction must be accurate as to where an earthquake will occur, when it will occur, and at what magnitude it will be so that people can evacuate. An unnecessary evacuation is expensive and causes people not to believe authorities the next time an evacuation is ordered.
Where an earthquake will occur is the easiest feature to predict. Scientists know that earthquakes take place at plate boundaries and tend to happen where they’ve occurred before. Earthquake-prone communities should always be prepared for an earthquake. These communities can implement building codes to make structures earthquake safe.
When an earthquake will occur is much more difficult to predict. Since stress on a fault builds up at the same rate over time, earthquakes should occur at regular intervals. But so far scientists cannot predict when quakes will occur even to within a few years. Signs sometimes come before a large earthquake. Small quakes, called foreshocks, sometimes occur a few seconds to a few weeks before a major quake. However, many earthquakes do not have foreshocks and small earthquakes are not necessarily followed by a large earthquake. Often, the rocks around a fault will dilate as microfractures form.
Ground tilting, caused by the buildup of stress in the rocks, may precede a large earthquake, but not always. Water levels in wells fluctuate as water moves into or out of fractures before an earthquake. This is also an uncertain predictor of large earthquakes. The relative arrival times of P-waves and S-waves also decreases just before an earthquake occurs.
Folklore tells of animals behaving erratically just before an earthquake. Mostly these anecdotes are told after the earthquake. If indeed animals sense danger from earthquakes or tsunami, scientists do not know what it is they could be sensing, but they would like to find out.
The geology of California underlies the state’s wealth of natural resources as well as its natural hazards. This video explores the enormous diversity of California’s geology, (57:50).
DAMAGE FROM EARTHQUAKES
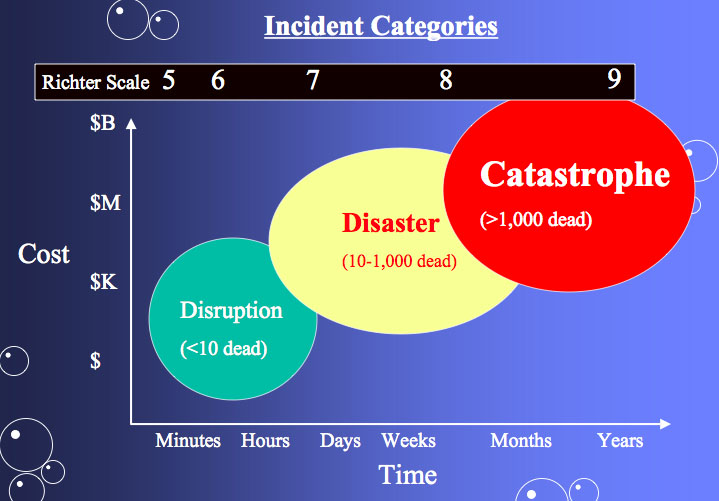
Earthquakes are natural disasters that cause enormous amounts of damage, second only to hurricanes. Earthquake-safe construction techniques, securing heavy objects, and preparing an emergency kit are among the precautions people can take to minimize damage.
Earthquakes kill people and cause property damage. However, the ground shaking almost never kills people, and the ground does not swallow someone up. The damage depends somewhat on the earthquake size but mostly on the quality of structures. Structures falling on people injure and kill them. More damage is done and more people are killed by the fires that follow an earthquake than the earthquake itself.
There are a variety of ways earthquakes become deadly. Probably the greatest influence is population density. The magnitude 9.2 Great Alaska Earthquake, near Anchorage, of 1964 resulted in only 131 deaths. At the time few people lived in the area. Oddly enough, size doesn’t matter. Only about 2,000 people died in the 1960 Great Chilean earthquake, the largest earthquake ever recorded. The Indian Ocean earthquake of 2004 was one of the largest ever, but most of the 230,000 fatalities were caused by the tsunami, not the earthquake itself.
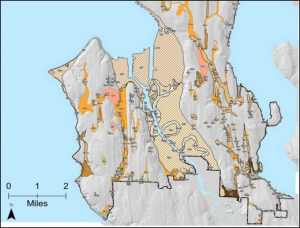
Ground type is an important factor in earthquake damage. Solid bedrock vibrates less than soft sediments so there is less damage on bedrock. Sediments that are saturated with water under go liquefaction and become like quicksand. This will have dangerous implications for Salt Lake City and the Wasatch Front in Utah. Much of the Wasatch Front is loose soil, left over from the remnants of Lake Bonneville. Along the middle of the two mountain ranges, between the Wasatch Mountains and the Oquirrh Mountains, is the Jordan River which flows from Utah Lake northward to the Great Salt Lake. Near the river and surrounding floodplain, the water table is near the surface. When the ground shakes, the water near the surface shifts upward and over-saturates the soil making it extremely unstable.
EARTHQUAKE-SAFE STRUCTURES
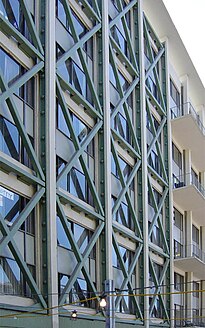
Construction is a large factor in what happens during an earthquake. For example, many more people died in the 1988 Armenia earthquake where people live in mud houses than in the 1989 earthquake in Loma Prieta. Most buildings in California’s earthquake country are designed to be earthquake safe.
The key to earthquake safety are the structures we live in. It is often said that earthquakes don’t kill people, it’s the buildings that kill people. But the reason why not all buildings are not built to withstand earthquakes is cost. More sturdy structures are much more expensive to build, so communities must weigh how great the hazard is, what different building strategies cost, and make an informed decision. But this is the crucial factor in earthquake safety. Let’s compare Salt Lake City, UT and Port-au-Prince, Haiti. When Salt Lake City has it’s expected 7.0 magnitude earthquake, worst case scenario is that 3,000-4,000 will die. But on January 12, 2010, Haiti experienced a 7.0 magnitude earthquake that killed nearly 300,000 people. The difference – building codes.
Skyscrapers and other large structures built on soft ground must be anchored to bedrock, even if it lies hundreds of meters below the ground surface. Larger buildings must sway, but not so much that they touch nearby buildings. Counterweights and diagonal steel beams are used to hold down sway. Large buildings can also be placed on rollers so that they move with the ground.
Earthquake prone areas should have building codes that require the use of correct building materials. Houses should also be built with wood and steel rather than brick and stone because they need to be able to bend and sway. New buildings should be built on layers of steel and rubber to absorb the shock of the waves. Connections, such as where the walls meet the foundation, must be made strong to withstand the shaking. In a multi-story building, the first story must be well supported.
To make older buildings more earthquake safe, retrofitting with steel or wood can reinforce a building’s structure and its connections. Elevated freeways and bridges can also be retrofitted so that they do not collapse.
Fires often cause more damage than the earthquake. Fires start because seismic waves rupture gas and electrical lines, and breaks in water mains make it difficult to fight the fires. Builders zigzag pipes so that they bend and flex when the ground shakes. In San Francisco, water and gas pipelines are separated by valves so that areas can be isolated if one segment breaks.
HUMAN-INDUCED EARTHQUAKES
Can humans create earthquakes? Maybe not intentionally, but the answer is yes and here is why. If a water reservoir is built on top of an active fault line, the water may actually lubricate the fault and weaken the stress built up within it. This may either create a series of small earthquakes or potentially create a large earthquake. Also the shear weight of the reservoir’s water can weaken the bedrock causing it to fracture. Then the obvious concern is if the dam fails. Earthquakes can also be generated if humans inject other fluids into a fault such as sewage or chemical waste. Finally, nuclear explosions can trigger earthquakes. In fact, one way to determine if a nation has tested a nuclear bomb is by monitoring the earthquakes and energy released by the explosion.
VOLCANOES: LOCATIONS
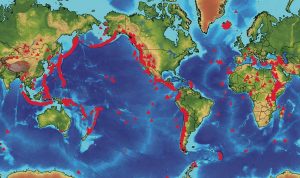
VOLCANOES ALONG CONVERGENT PLATE BOUNDARIES
Volcanoes are a vibrant manifestation of plate tectonics processes. Volcanoes are common along convergent and divergent plate boundaries, but are also found within lithospheric plates away from plate boundaries. Wherever mantle is able to melt, volcanoes may be the result.
Volcanoes erupt because mantle rock melts. The first stage in creating a volcano is when mantle rock begins to melt because of extremely high temperatures, lithospheric pressure lowers, or water is added. Along subducting plate boundaries, the crust heats up as it sinks into the mantle. Also, ocean water is mixed in with the sediments lying on top of the subducting plate. This water lowers the melting point of the mantle material, which increases melting. Volcanoes at convergent plate boundaries are found all along the Pacific Ocean basin, primarily at the edges of the Pacific, Cocos, and Nazca plates.
Large earthquakes are extremely common along convergent plate boundaries. Since the Pacific Ocean is rimmed by convergent and transform boundaries, about 80% of all earthquakes strike around the Pacific Ocean basin and is why the region is called the Ring of Fire. A description of the Pacific Ring of Fire along western North America is below:
- Subduction at the Middle American Trench creates volcanoes in Central America.
- The San Andreas Fault is a transform boundary.
- Subduction of the Juan de Fuca plate beneath the North American plate creates the Cascade volcanoes like Mount St. Helens, Mount Rainer, Mount Hood and more.
- Subduction of the Pacific plate beneath the North American plate in the north creates the long chain of the Aleutian Islands volcanoes near Alaska.
This incredible explosive eruption of Mount Vesuvius in Italy in A.D. 79 is an example of a composite volcano that forms as the result of a convergent plate boundary.
VOLCANOES ALONG DIVERGENT PLATE BOUNDARIES

Why does melting occur at divergent plate boundaries? Hot mantle rock rises where the plates are moving apart. This releases pressure on the mantle, which lowers its melting temperature allowing lava to erupt through long cracks or fissures. Scientists have captured incredible footage of “Deepest Ocean Eruption Ever Filmed” and other undersea volcanoes. Volcanoes erupt at mid-ocean ridges, such as the Mid-Atlantic ridge, where seafloor spreading creates new seafloor in the rift valleys. Where a hotspot is located along the ridge, such as at Iceland, volcanoes grow high enough to create islands. Eruptions are found at divergent plate boundaries as continents break apart such as the East African Rift between the African and Arabian plates and the Great Basin and Range in the western United States. But those volcanoes of the ladder are now extinct.
VOLCANIC HOTSPOTS
Although most volcanoes are found at convergent or divergent plate boundaries, intraplate volcanoes are found in the middle of a tectonic plate. The Hawaiian Islands are the exposed peaks of a great chain of volcanoes that lie on the Pacific plate. These islands are in the middle of the Pacific plate. The youngest island sits directly above a column of hot rock called a mantle plume. As the plume rises through the mantle, pressure is released and mantle melts to create a hotspot.
Earth is home to about 50 known hot spots. Most of these are in the oceans because they are better able to penetrate oceanic lithosphere to create volcanoes. The hotspots that are known beneath continents are extremely large, such as Yellowstone. The video on the right is of the hot spot beneath Hawaii, the origin of the voluminous lava produced by the shield volcano Kilauea. There are several key indicators to determine a hot spot from island arc volcanoes. At island arcs, the volcanoes are all about the same age. By contrast, at hotspots the volcanoes are youngest at one end of the chain and oldest at the other.
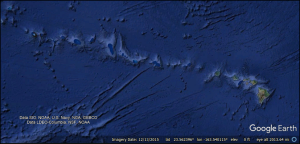
LOCATION AND MAGMA COMPOSITION
It should first be noted that magma is molten material inside the earth, whereas lava is molten material on the surface of the earth. The reason for the distinction is because lava can cool quickly from the air and solidify into rock rapidly, whereas magma may never reach the earth’s surface. Volcanoes do not always erupt in the same way. Each volcanic eruption is unique, differing in size, style, and composition of erupted material. One key to what makes the eruption unique is the chemical composition of the magma that feeds a volcano, which determines (1) the eruption style, (2) the type of volcanic cone that forms, and (3) the composition of rocks that are found at the volcano.
Different minerals within a rocks melt at different temperatures and the amount of partial melting and the composition of the original rock determine the composition of the magma. Magma collects in magma chambers in the crust at 160 kilometers (100 miles) beneath the surface of a volcano.
The words that describe composition of igneous rocks also describe magma composition. Mafic magmas are low in silica and contain more dark, magnesium and iron rich mafic minerals, such as olivine and pyroxene. Felsic magmas are higher in silica and contain lighter colored minerals such as quartz and orthoclase feldspar. The higher the amount of silica in the magma, the higher is its viscosity. Viscosity is a liquid’s resistance to flow.
Viscosity determines what the magma will do. Mafic magma is not viscous and will flow easily to the surface. Felsic magma is viscous and does not flow easily. Most felsic magma will stay deeper in the crust and will cool to form igneous intrusive rocks such as granite and granodiorite. If felsic magma rises into a magma chamber, it may be too viscous to move and so it gets stuck. Dissolved gases become trapped by thick magma and the magma chamber begins to build pressure.
EXPLOSIVE ERUPTIONS
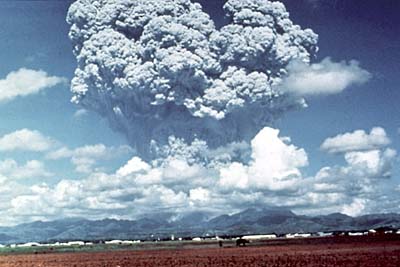
The type of magma in the chamber determines the type of volcanic eruption. A large explosive eruption creates even more devastation than the force of the atom bomb dropped on Nagasaki at the end of World War II in which more than 40,000 people died. A large explosive volcanic eruption is 10,000 times as powerful. Felsic magmas erupt explosively because of hot, gas-rich magma churning within its chamber. The pressure becomes so great that the magma eventually breaks the seal and explodes, just like when a cork is released from a bottle of champagne. Magma, rock, and ash burst upward in an enormous explosion creating volcanic ash called tephra. It should be noted that when looked under a microscope, the volcanic “ash” is actual microscopic shards of glass. That is why it is so dangerous to inhale the air following an eruption.
Scorching hot tephra, ash, and gas may speed down the volcano’s slopes at 700 km/h (450 mph) as a pyroclastic flow. Pyroclastic flows knock down everything in their path. The temperature inside a pyroclastic flow may be as high as 1,000°C (1,800° F).
Prior to the Mount St. Helens eruption in 1980, the Lassen Peak eruption on May 22, 1915, was the most recent Cascades eruption. A column of ash and gas shot 30,000 feet into the air. This triggered a high-speed pyroclastic flow, which melted snow and created a volcanic mudflow known as a lahar. Lassen Peak currently has geothermal activity and could erupt explosively again. Mt. Shasta, the other active volcano in California, erupts every 600 to 800 years. An eruption would most likely create a large pyroclastic flow, and probably a lahar. Of course, Mt. Shasta could explode and collapse like Mt. Mazama in Oregon.
Volcanic gases can form poisonous and invisible clouds in the atmosphere that could contribute to environmental problems such as acid rain and ozone destruction. Particles of dust and ash may stay in the atmosphere for years, disrupting weather patterns and blocking sunlight.
EFFUSIVE ERUPTIONS
Mafic magma creates gentler effusive eruptions. Although the pressure builds enough for the magma to erupt, it does not erupt with the same explosive force as felsic magma. People can usually be evacuated before an effusive eruption, so they are much less deadly. Magma pushes toward the surface through fissures and reaches the surface through volcanic vents. Click here to view a lava stream within the vent of a Hawaiian volcano using a thermal camera. Low-viscosity lava flows down mountainsides. Differences in composition and where the lavas erupt result in lava types like a ropy form pahoehoe and a chunky form called aa. Although effusive eruptions rarely kill anyone, they can be destructive. Even when people know that a lava flow is approaching, there is not much anyone can do to stop it from destroying a building, road, or infrastructure.
PREDICTING ERUPTIONS
Volcanologists attempt to forecast volcanic eruptions, but this has proven to be nearly as difficult as predicting an earthquake. Many pieces of evidence can mean that a volcano is about to erupt, but the time and magnitude of the eruption are difficult to pin down. This evidence includes the history of previous volcanic activity, earthquakes, slope deformation, and gas emissions.
HISTORY OF VOLCANIC ACTIVITY
A volcano’s history, how long since its last eruption and the recurrence interval between its previous eruptions, is a good first step to predicting eruptions. If the volcano is considered active, it is currently erupting or shows signs of erupting soon. A dormant volcano means there is no current activity, but it has erupted recently. Finally, an extinct volcano means their is no activity and will probably not erupt again. Active and dormant volcanoes are heavily monitored, especially in populated areas.
EARTHQUAKES
Moving magma shakes the ground, so the number and size of earthquakes increases before an eruption. A volcano that is about to erupt may produce a sequence of earthquakes. Scientists use seismographs that record the length and strength of each earthquake to try to determine if an eruption is imminent.
Magma and gas can push the volcano’s slope upward. Most ground deformation is subtle and can only be detected by tiltmeters, which are instruments that measure the angle of the slope of a volcano. But ground swelling may sometimes create huge changes in the shape of a volcano. Mount St. Helens grew a bulge on its north side before its 1980 eruption. Ground swelling may also increase rockfalls and landslides.
GAS EMISSIONS
Gases may be able to escape a volcano before magma reaches the surface. Scientists measure gas emissions in vents on or around the volcano. Gases, such as sulfur dioxide (SO2), carbon dioxide (CO2), hydrochloric acid (HCl), and even water vapor can be measured at the site or, in some cases, from a distance using satellites. The amounts of gases and their ratios are calculated to help predict eruptions.
REMOTE MONITORING and DEFORMATION
Some gases can be monitored using satellite technology. Satellites also monitor temperature readings and deformation. As technology improves, scientists are better able to detect changes in a volcano accurately and safely.
Since volcanologists are usually uncertain about an eruption, officials may not know whether to require an evacuation. If people are evacuated and the eruption doesn’t happen, the people will be displeased and less likely to evacuate the next time there is a threat of an eruption. The costs of disrupting business are great. However, scientists continue to work to improve the accuracy of their predictions.
TYPES OF VOLCANOES

A volcano is a vent through which molten rock and gas escape from a magma chamber and they can differ in height, shape, and slope steepness. Some volcanoes are tall cones and others are just cracks in the ground. As you might expect, the shape of a volcano is related to the composition of its magma.
COMPOSITE (STRATO) VOLCANOES
Composite volcanoes are some of the most dangerous volcanoes on the planet. They tend to occur along oceanic-to-oceanic or oceanic-to-continental boundaries because of subduction zones. They tend to be made of felsic to intermediate rock and the viscosity of the lava means that eruptions tend to be explosive. The viscous lava cannot travel far down the sides of the volcano before it solidifies, which creates the steep slopes of a composite volcano. Viscosity also causes some eruptions to explode as ash and small rocks. The volcano is constructed layer by layer, as ash and lava solidify, one upon the other and are sometimes called stratovolcanoes or andesite volcanoes. The result is the classic cone shape of composite volcanoes. Examples of composite volcanoes include Mount St. Helens, Mount Rainer, Mount Shasta, Mount Hood, and Mount Pinatubo. Here’s a great time-lapse of Mount St. Helens from NASA’s Earth Observatory from 1979 to 2013.Sometimes composite volcanoes and other violent volcanoes can erupt so violently that they sometimes collapse in on themselves or actually blow themselves up to produce calderas. One of the most powerful volcanoes in the world – Yellowstone – is a massive caldera that has collapsed several times. Sometimes these calderas can fill up with water to produce beautiful lakes such as Mount Mazama (Crater Lake), in Oregon.
SHIELD VOLCANOES
Shield volcanoes get their name from their shape. Although shield volcanoes are not steep, they may be very large. In fact, Mauna Loa, Hawaii is the tallest mountain in the world. From sea level, Mount Everest is the tallest, but when you consider from the ocean floor to the top of the island, Mauna Loa wins. Shield volcanoes are common at spreading centers or intraplate hot spots.
The lava that creates shield volcanoes is fluid and flows easily and creates the shield shape. Shield volcanoes are built by many layers over time and the layers are usually of very similar composition. The low viscosity also means that shield eruptions are non-explosive. Eruptions tend to be mild in comparison to other volcanoes, but lava flows can destroy property and vegetation. The low viscosity magma can flow not only on the surface as lava, but also underground in lava tubes. The most well known shield volcano is Hawaii. There are two types of lava flows, pahoehoe which is a ropy type of lava that flows easily (low viscosity). The other type is called aa and is a blocky type of lava and has a higher viscosity and does not like to flow well.

CINDER CONES
Cinder cones are the most common type of volcano. A cinder cone has a cone shape, but is much smaller than a composite volcano. Cinder cones rarely reach 300 meters in height but they have steep sides. Cinder cones grow rapidly, usually from a single eruption cycle. Cinder cones are composed of small fragments of rock, such as pumice, piled on top of one another. The rock shoots up in the air and doesn’t fall far from the vent. The exact composition of a cinder cone depends on the composition of the lava ejected from the volcano. Cinder cones usually have a crater at the summit. Cinder cones are often found near larger volcanoes.
SUPERVOLCANOES
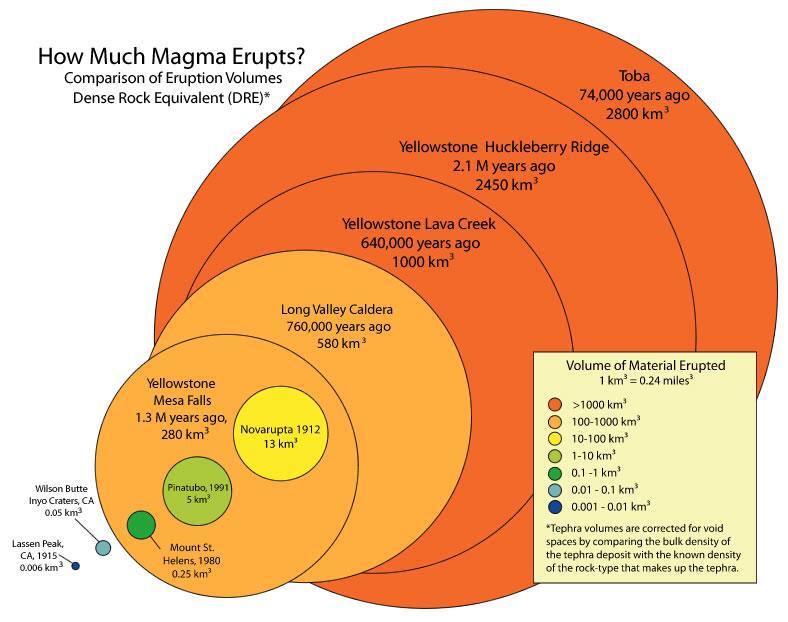
Supervolcano eruptions are extremely rare in Earth history. It’s a good thing because they are unimaginably large. A supervolcano must erupt more than 1,000 cubic km (240 cubic miles) of material, compared with 1.2 km3 for Mount St. Helens or 25 km3 for Mount Pinatubo, a large eruption in the Philippines in 1991. Not surprisingly, supervolcanoes are the most dangerous type of volcano. Supervolcanoes are a fairly new idea in volcanology. The exact cause of supervolcano eruptions is still debated, however, scientists think that a very large magma chamber erupts entirely in one catastrophic explosion. This creates a huge hole or caldera into which the surface collapses.
The largest supervolcano in North America is beneath Yellowstone National Park in Wyoming. Yellowstone sits above a hotspot that has erupted catastrophically three times: 2.1 million, 1.3 million, and 640,000 years ago. Yellowstone has produced many smaller (but still enormous) eruptions more recently. Fortunately, current activity at Yellowstone is limited to the region’s famous geysers.
Long Valley Caldera, south of Mono Lake in California, is the second largest supervolcano in North America. Long Valley had an extremely hot and explosive rhyolite explosion about 700,000 years ago. An earthquake swarm in 1980 alerted geologists to the possibility of a future eruption, but the quakes have since calmed down.
A supervolcano could change life on Earth as we know it. Ash could block sunlight so much that photosynthesis would be reduced and global temperatures would plummet. Volcanic eruptions could have contributed to some of the mass extinctions in our planet’s history. No one knows when the next super eruption will be.
VOLCANIC LANDFORMS AND GEOTHERMAL ACTIVITY
VOLCANIC LANDFORMS AND VENTS
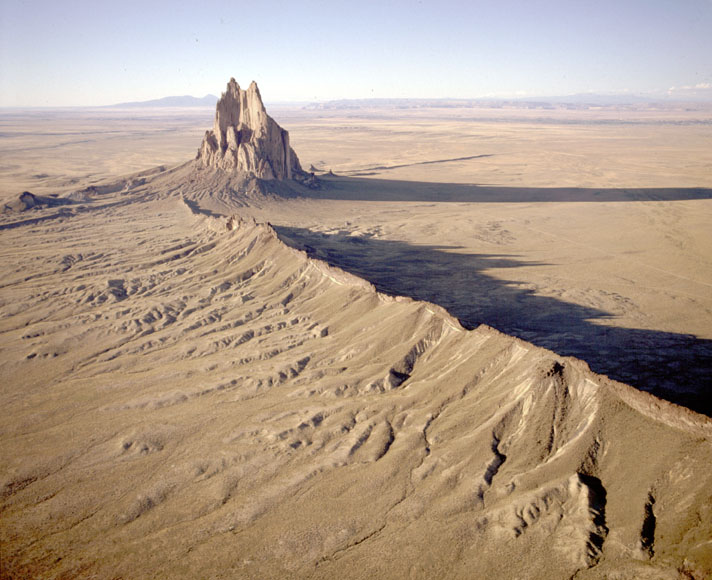
Volcanoes are associated with many types of landforms. The landforms vary with the composition of the magma that created them. Hot springs and geysers are also examples of surface features related to volcanic activity.
The most obvious landforms created by lava are volcanoes, most commonly as cinder cones, composite volcanoes, and shield volcanoes or eruptions that take place through fissures. The eruptions that created the entire ocean floor are essentially fissure eruptions. Magma intrusions ALSO can create landforms. The image on the right is of Shiprock in New Mexico, which is the neck of an old volcano that has eroded away.
A lava plateau forms when large amounts of fluid lava flows over an extensive area. When the lava solidifies, it creates a large, flat surface of igneous rock. Lava creates new land as it solidifies on the coast or emerges from beneath the water. Over time the eruptions can create whole islands. The Hawaiian Islands are formed from shield volcano eruptions that have grown over the last 5 million years.
HOT SPRINGS AND GEYSERS
Water sometimes comes into contact with hot rock. The water may emerge at the surface as either a hot spring or a geyser. Water heated below ground that rises through a crack to the surface creates a hot spring. The water in hot springs may reach temperatures in the hundreds of degrees Celsius beneath the surface, although most hot springs are much cooler.
VOLCANOES: HAZARDS AND BENEFITS
There are several hazards that volcanic activity can produce.
- Eruption clouds occur when massive quantities of ash is ejected into the atmosphere where it can reach heights of 50,000 feet. Eruption clouds have proven to be very dangerous for aviation jets because the ash can shut down the engines. The ash cloud can also be very hazardous in terms of air pollution.
- Lahars are volcanic mudflows. Lahars are very dangerous because they do not require a volcanic eruption yet can travel hundreds of miles. All that is required is loose pyroclastic material on the volcano that mixes with precipitation or melting snow.
- Lava flows are layers of molten rock that flow over the surface, later cooling and solidifying.
- Lava bombs are large chunks of pyroclastic material ejected from a volcano. Larger pyroclastic material is called blocks.
- Pyroclastic flows are some of the most dangerous hazards caused by composite volcanoes. Pyroclastic flows are superheated clouds of pyroclastic material (e.g. hot rock and tephra) ranging in size from small rocks to the size of houses that are over 1,000 degrees F traveling down a mountain at speeds up to 100 mph.
- Tephra (or volcanic ash) is fine particles of pyroclastic material that can be carried thousands of miles away by prevailing winds. Regions hundreds of miles away could suffer collapsed buildings is the falling ash accumulates enough. Tephra can also cool the entire planet if enough is ejected into the atmosphere.
- Poisonous gases such as carbon dioxide, carbon monoxide, and sulfur dioxide can travel down a volcano and asphyxiate (suffocating) wildlife and humans. In 1986, an invisible cloud of carbon dioxide traveled down a volcano in Africa asphyxiating 1,742 people and 3,000 cattle.
There are actually many benefits to volcanic activity. One of the major benefits is the fact that volcanic activity can create very fertile soil for agriculture. The problem is that many civilizations developed near volcanoes for this reason – with sometimes deadly effects. Volcanic activity can also create many mineral resources such as gold, sliver, nickel, copper, and lead. Volcanic rock is often used for landscaping, tile, and cement.
Some of the most amazing landscapes are near volcanoes. This is because volcanic activity builds land creating breathtaking scenery. So volcanoes are economically vital for many regions because of the recreational activity and tourism they bring.
Finally, a new but important trend is geothermal power. The heat generated by volcanoes can create electricity to power civilization. Geothermal power is a completely renewable resource free of pollution and energy dependency on fossil fuels. Iceland – the surface manifestation of the mid-Atlantic ridge – has a goal of powering the entire nation on geothermal energy. Geothermal energy is also being used in California, Kilauea, Hawaii, and now Utah.